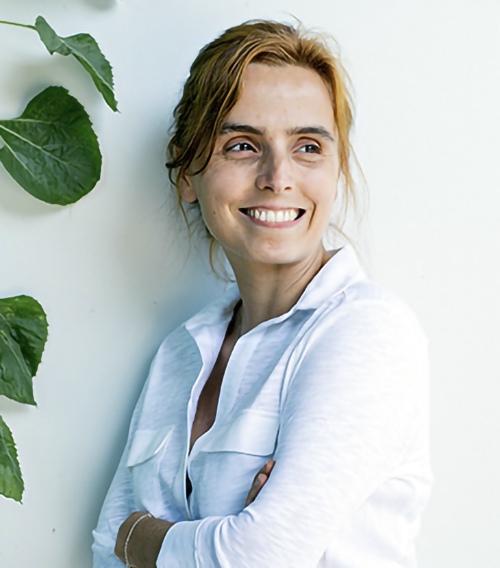
Nature's Hidden Artistry: Unveiling the Secret Blueprints of Flower Patterns
In a groundbreaking study published in Science Advances, scientists at the University of Cambridge’s Sainsbury Laboratory have made a remarkable discovery in the world of plant biology. Led by Dr. Edwige Moyroud, the research team has uncovered an invisible “pre-pattern” that shapes the iconic bullseye designs on hibiscus petals. This finding reveals nature’s equivalent of a “paint-by-numbers” system, active long before any visible colors emerge, and offers new insights into the intricate process of floral pattern formation.
The study demonstrates that these early developmental blueprints play a crucial role in attracting pollinators, particularly bees. Surprisingly, bees show a clear preference for larger bullseyes, moving between flowers with bigger patterns 25% faster. This discovery highlights the delicate interplay between plant evolution and pollinator behavior. By comparing different hibiscus species, including the critically endangered Hibiscus richardsonii with its small bullseye and the more common Hibiscus trionum with a medium-sized bullseye, the scientists revealed how these subtle early patterns can have far-reaching ecological consequences.
This research not only advances our understanding of how flowers develop their intricate patterns but also opens new avenues for exploring biodiversity and plant-pollinator relationships. The insights gained from this study could prove invaluable for conservation efforts, potentially offering new strategies to protect endangered plant species and their pollinators. As we delve deeper into Dr. Moyroud’s fascinating work, we’ll explore how these microscopic masterpieces of nature shape the vibrant world of flowers and the creatures that depend on them.
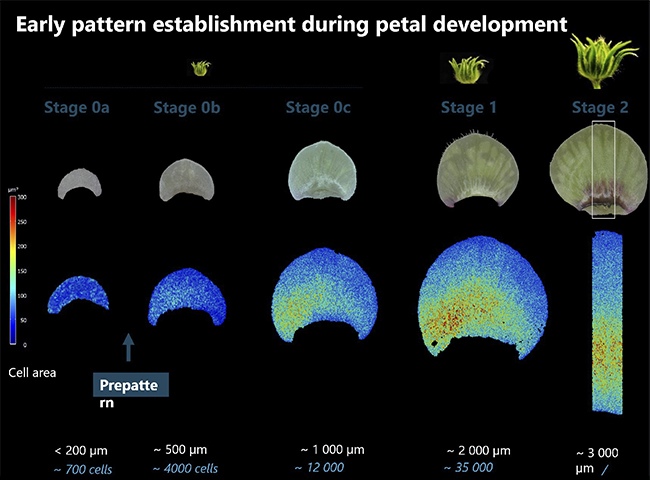
Q: Your recent study highlights the existence of an invisible pre-pattern in hibiscus flowers. Could you elaborate on how this pre-pattern is formed and what tools you used to detect it during early petal development?
A: We refer to it as a “pre-pattern” because it’s an underlying blueprint that isn’t immediately visible when the flower opens. This pre-pattern is crucial in creating the conspicuous differences in color, cell shape, and texture that make the two parts of the petal look so distinct.
Researchers have long tried to understand how these patterns are formed because they play a vital role in mediating communication between plants and pollinators. Flowering plants dominate the Earth today, largely because flowers are a remarkable evolutionary innovation that enhances reproductive efficiency. While there are non-flowering plants like conifers (such as Christmas trees), flowering plants have largely overtaken them due to these advantages.
Historical records show that as insects diversified, flowering plants did as well. Petals are often the most visually prominent part of the flower—ask a child to draw a flower, and they’ll usually sketch the petals, overlooking other important parts because they’re less visible.
Recent studies have revealed that these patterns serve additional functions. For instance, they help flowers resist desiccation in dry environments and protect against high levels of UV radiation, much like sunscreen. Recognizing their importance, we set out to understand how these patterns form. However, progress had been slow because researchers were focusing on the wrong developmental stage.
Our study discovered that these patterns form very early in development, at a stage when petals appear uniform and green to the naked eye. Traditionally, scientists studied petal development by examining buds at various growth stages—imagine tiny buds forming and growing on the plant. They would collect these buds, examine the petals, and eventually see the patterns appear on the surface. But by that point, the crucial processes establishing the pattern had already occurred.
To truly understand pattern formation, we needed to investigate these earlier stages when no visible differences are apparent. We used powerful microscopes to image the outline of every cell, analyzing their geometry and size. Although the cells seemed identical superficially, we detected subtle differences in symmetry and size, particularly around the boundary separating the two parts of the petal where the pattern emerges.
I like to use the analogy of a “paint-by-number” canvas. As a child, I enjoyed coloring these canvases that started as a black-and-white outline divided into numbered sections, each corresponding to a specific color. Even though the canvas initially appeared uniform, the underlying divisions guided the final image. Similarly, during early petal development, cells are assigned positions and identities that determine how they will differentiate later on—even if we can’t see any differences on the surface yet.
By examining the geometry and behavior of cells at this early stage, we discovered that the division of the petal into different regions happens much sooner than previously thought. While these differences aren’t visible to the naked eye, our microscopic analysis revealed the pre-pattern that sets the stage for the striking patterns we see when the flower blooms.

Q: The concept of a ‘paint-by-numbers’ system for petal development is fascinating. How did you identify that specific regions of the petal were pre-determined to develop into the bullseye pattern?
A: Our discovery was largely made possible by advanced imaging technology. We began by dissecting flower buds at various stages of development, working backwards from open flowers to increasingly smaller buds. Our goal was to find the earliest stage where the petals were still completely green with no visible pattern.
We used a fluorescent protein that highlights cell outlines, allowing us to visualize the cellular structure under a fluorescent microscope. This technique gave us a view similar to the outlines on a paint-by-numbers canvas.
The breakthrough came when we examined these early-stage, seemingly patternless petals. Despite the lack of visible pigmentation, we noticed that the cells weren’t uniform in size. Crucially, we observed larger cells precisely where the boundary of the bullseye pattern would later emerge.
This cellular differentiation was invisible to the naked eye, but our imaging technique revealed that the pattern was essentially already “drawn” at the cellular level. It was like discovering that nature had sketched out the design long before applying any “paint.”
This finding was only possible due to technological advancements that allow us to see cellular details that were previously unobservable. It demonstrates how new tools can reveal hidden processes in nature, even in phenomena we thought we understood.
Q: Can you describe the process by which you compared different hibiscus species, such as Hibiscus trionum and Hibiscus richardsonii, to explore the role of bullseye size in attracting pollinators?
A: Certainly! Comparing open flowers is straightforward—we separate the petals, scan them, and use automated software to detect different regions and measure pigmentation levels, efficiently quantifying the bullseye sizes.
The challenge arises when imaging the pre-patterns, which are invisible to the naked eye and exist in extremely small petals (about 2 millimeters long). We carefully dissect these tiny petals, often using dissecting microscopes for precision. After dissection, we place the petals under advanced microscopes that use lasers and fluorescence to detect cell outlines.
We employ chemical dyes that make cell walls glow under the microscope, revealing the “paint-by-number” design of the pre-pattern. Since manual measurement is impractical due to thousands of cells, we use sophisticated software to automatically identify cell outlines and measure dimensions like length, geometry, and size. While the software requires some corrections, it significantly speeds up the process.
By combining these methods, we effectively compare the pre-patterns between different hibiscus species, allowing us to explore how variations in bullseye size may influence pollinator attraction.
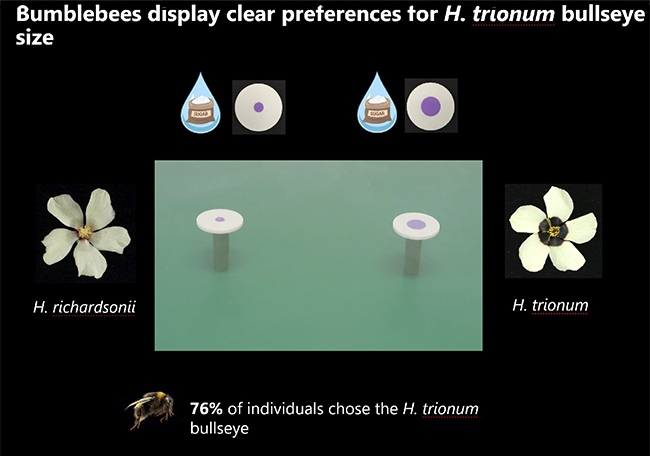
Q: Your study found that bees prefer larger bullseyes and fly 25% faster between flowers with bigger patterns. How do these findings enhance our understanding of plant-pollinator interactions, and what implications could this have for plant reproduction and survival?
A: Our findings reveal a complex relationship between plants and pollinators. Despite bees preferring larger bullseyes, we observed an evolutionary trend in hibiscus plants towards smaller bullseyes. This contradiction suggests several possibilities:
1. Different pollinators may prefer smaller bullseyes, potentially leading to speciation.
2. Plants with smaller bullseyes might compensate in other ways:
*Some flowers remain open longer, increasing pollination chances.
*Others produce more nectar, attracting bees despite less visual appeal.
These strategies highlight the diverse ways plants ensure reproduction and survival. From a conservation perspective, understanding these preferences can help us support threatened pollinator populations. For instance, introducing plants with large bullseyes could aid declining bumblebee colonies by making foraging more efficient.
Overall, these findings emphasize the complexity of plant-pollinator relationships and their importance in maintaining biodiversity.
Q: Given that Hibiscus richardsonii is critically endangered, do you think its smaller bullseye pattern could be a factor in its declining population? How might this discovery contribute to conservation efforts for endangered species?
A: Yes, the smaller bullseye pattern of Hibiscus richardsonii could be contributing to its decline if pollinators prefer flowers with larger bullseyes. If a competing species with a larger bullseye was introduced, it might attract more pollinators, leading to reduced reproduction for H. richardsonii.
We’ve conducted hand-pollination experiments showing that H. richardsonii can cross-pollinate with species having larger bullseyes, indicating no physiological barriers. Both species coexist in parts of New Zealand, so studying whether they naturally cross or are visited by different pollinators is important.
To minimize human interference, we’re planning to use GoPro cameras to record pollinator interactions with these flowers. Understanding what attracts different pollinators is crucial for conservation efforts.
By identifying the genes responsible for bullseye size, we could potentially modify H. richardsonii to have a larger bullseye, making it more attractive to pollinators and aiding its survival. While this approach is speculative and subject to regulations, it offers a possible conservation strategy.
Beyond this, our research enhances understanding of how cells make developmental decisions, which could have broader implications in biology and medicine.
Q: Have you mapped out the entire genome for these flowers?
A: We’ve started identifying some of the important genes, although we didn’t include this information in our recent paper. Another paper we’re working on identifies some of the genes that control the size of the pigmentation in Hibiscus richardsonii. In our experiments, we either disrupted these genes in Hibiscus trionum, which resulted in a smaller bullseye pattern, or reintroduced them into Hibiscus richardsonii, causing the bullseye to enlarge. This means we’re beginning to understand how to manipulate these traits. While there are likely many genes and networks involved, we’re excited to be making progress in pinpointing the specific genes responsible.
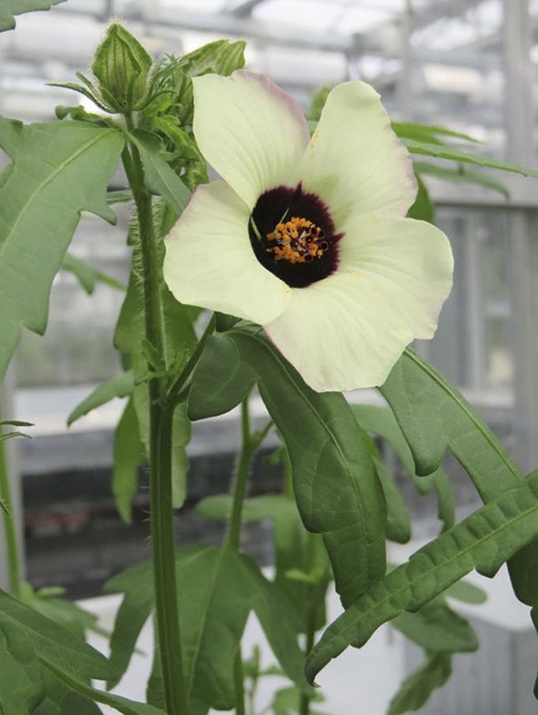
Q: The idea that pre-patterning could give plants an evolutionary advantage is intriguing. How do you think these mechanisms evolved, and could this pre-patterning process be found in other flower species or even plant organs like leaves?
A: It’s one of those phenomena where, once you notice it, you start seeing it everywhere. Flowering plants appeared roughly 150 million years ago, and although we don’t fully understand how pre-patterning works, there’s a theory that all parts of a flower are modified leaves. Since leaves existed before flowers, petals may have evolved from leaves by acquiring the ability to become colorful and change shape.
We believe that some of the processes petals use for their patterning are recycled or modified from those already present in leaves. If you examine a leaf, you might notice subtle patterns—though they’re less obvious because most leaves lack prominent pigmentation. The shape of a leaf varies from base to tip, and under a microscope, you can observe different cell behaviors.
So, we think that the pre-patterning we’re studying isn’t exclusive to petals but also applies to all lateral organs like leaves and stamens. While the processes might not be exactly the same, there’s likely a core mechanism that’s shared, with each organ evolving its own specific features. We need to conduct further research to determine how much of this is true, but it’s an exciting possibility that could extend our understanding of plant development across various species and organs.
Q: You mentioned that the pre-pattern begins before the petal shows any visible color. Could you explain how these early developmental processes relate to the later stages of growth, where visible patterns emerge?
A: Certainly! To create a pattern in the petal, imagine the epidermis—a layer of cells all adjacent to each other. Although these cells are neighbors and part of the same tissue, they need to become very different during development.
In the case of hibiscus petals, the cells at the top form tiny cones that grow outward from the petal surface. These cells remain white and completely smooth. In contrast, the cells at the base of the petal produce purple pigment. They grow by elongating into long rectangles, don’t protrude like the cones, and develop fine striations that can scatter light.
Despite being side by side, these cells follow very different developmental paths. We believe this divergence is guided by the pre-pattern boundary—a sort of invisible line acting like a wall. Cells recognize their position relative to this boundary: if they’re above the line, they follow one developmental trajectory; if they’re below it, they follow another. This compartmentalization allows for distinct behaviors in adjacent cells.
Think of it like dividing a cake so that each half can have different flavors. Without the division, both halves would be the same. Similarly, the pre-pattern boundary is crucial because it enables cells to specialize before any visible patterns emerge. Although we can’t see this boundary, it’s fundamentally important to the final appearance of the petal.
It’s interesting to compare the pre-pattern to quiet individuals who work behind the scenes—they might not draw attention, but their contributions are vital. In the same way, the pre-pattern operates silently but has a profound impact on the flower’s development.
Q: Your research utilized artificial flower discs to mimic different bullseye dimensions. How did you design these experiments, and were you surprised by the bees’ preference for larger bullseyes?
A: As a geneticist and cell biologist, I wanted to ensure our experiments isolated the effect of bullseye size from other variables. Natural flowers might differ in scent, shape, or other unaccounted factors, making it difficult to attribute bee preferences solely to bullseye size.
To control for these variables, we created 3D-printed discs that mimicked the size of open flowers but varied only in bullseye proportions. We based these on real-world differences between Hibiscus trionum (large bullseye) and Hibiscus richardsonii (small bullseye).
I was impressed that bees could distinguish between these artificial patterns. However, we later reproduced the test with real flowers, expecting some compensatory factors might emerge. Surprisingly, the preference for larger bullseyes was even more pronounced with real flowers, with 8-9 out of 10 bees choosing H. trionum.
Our research suggests there might be an optimal bullseye-to-petal ratio across species, possibly due to evolutionary selection. However, plants can easily change bullseye size, which raises questions about why some produce smaller bullseyes.
This could be part of a complex trade-off. Floral patterns not only attract pollinators but also guide their behavior for effective pollination. For instance, without proper patterns, bees might access nectar without facilitating pollination.
These patterns serve dual roles: attracting pollinators to the flower and ensuring they interact with the flower correctly for successful pollination. This balance highlights the intricate relationships between plants and their pollinators.
We’re now exploring the limits of this preference, testing smaller differences in bullseye size and varying contrast levels. Interestingly, our survey of bullseye sizes across various species in botanical gardens suggests there might be an optimal bullseye-to-petal ratio, possibly resulting from evolutionary selection.
Q: Could you explain the role of computational modeling in your study and how it helped to simulate the development of petal patterns?
A: Computational modeling played a crucial role in our study by allowing us to simulate and visualize complex developmental processes that are beyond the capacity of our human brains to compute mentally. While we can intuitively imagine how a few cells might divide or elongate, a petal consists of thousands of cells, making it impossible to accurately predict the outcome without assistance.
Using computational models, we created virtual petals where we could rapidly run simulations of various cellular behaviors and genetic conditions. This approach is similar to how pilots use flight simulators to practice flying—it’s a safe and efficient way to test different scenarios. The computer can handle thousands of variables and conditions effortlessly, enabling us to explore a vast number of hypotheses quickly.
By simulating these experiments, we identified which factors had the most significant impact on petal pattern development. This process helped us pinpoint the most promising avenues for real-world experiments, saving us considerable time and resources. Instead of conducting numerous lengthy and potentially uninformative experiments, we could focus on those most likely to yield valuable insights. In summary, computational modeling allowed us to visualize complex processes, test a wide range of conditions efficiently, and prioritize the most informative experiments for our study.
Q: What were the challenges in studying such fine-scale petal development, particularly when dealing with very young petals less than 0.2 mm in size?
A: We faced several significant challenges in studying fine-scale petal development in such tiny petals.
The first challenge was the need for a suitable model organism. Traditionally, plant development studies use Arabidopsis thaliana, a small weed that has been a model plant for over 40 years. Laboratories worldwide have developed extensive resources for Arabidopsis, including its fully sequenced genome and protocols for gene manipulation. However, Arabidopsis produces very tiny flowers, self-pollinates, and lacks petal patterns that attract pollinators—there are no patterns on its petals. Therefore, it wasn’t suitable for studying how petal patterns attract pollinators.
We had to develop a new model system, so we searched for a species with the desired petal patterns that would also be practical for laboratory work. The ideal plant needed to grow quickly, be robust, produce lots of seeds, and allow for cross-pollination. Additionally, we had to develop methods to manipulate gene expression in this new plant, which was crucial for making it a good model organism. This process of finding and optimizing a new model plant was our first major challenge.
The second challenge involved imaging the petal development. The key to discovering the pre-pattern was using powerful microscopy to image cell behavior. However, no one had done this with petals before because petal development occurs inside closed buds, enclosed by green sepals, making them not readily accessible. We had to devise new methodologies to access these developing petals and establish imaging pipelines to study them effectively.
The final challenge was managing the massive amounts of data generated. Even though the petals are very small, they contain thousands of cells. Imaging these cells produced large datasets that required significant computational resources to process. We had to spend extensive computational time identifying all the cells and extracting meaningful data. While handling such large datasets increased our confidence in the results, it made image analysis time-consuming. To address this, we developed computational pipelines to manage and analyze the data efficiently.
These challenges highlight why this type of research hadn’t been done before—we needed to develop both a new model system using hibiscus and new methodologies for imaging and computational analysis.
Looking ahead, employing AI would be a valuable next step. AI could assist in identifying cellular behaviors from imaging datasets more efficiently. Additionally, we’d be interested in using AI to better understand animal behavior. For example, when we record bees visiting flowers and slow down the videos, we notice behaviors that aren’t apparent in real-time. AI could help analyze these recordings to uncover insights about bee behavior that we might otherwise miss.
Q: How might this research on flower pre-patterns impact other fields of biology, such as agricultural science or the study of ecological networks?
A: Our research on flower pre-patterns has potential impacts across several fields of biology:
1. Fundamental biology: Understanding how cells make decisions is a universal question in biology. This research could provide insights applicable to various fields, including human biology and cancer research, where cell decision-making is crucial.
2. Human health: The pigments we study, flavonoids, are known for their antioxidant properties and health benefits. By understanding how these molecules affect plant cells, we might gain insights into their effects on human health.
3. Agricultural science: a) Crop enhancement: Our research could lead to developing crops richer in beneficial compounds. For example, the “purple tomato” was engineered using similar principles. b) Pollination efficiency: Understanding floral patterns could help improve crop pollination. For instance, in the UK, field bean varieties bred for larger yields lost their black spot pattern, which attracts pollinators. Our research could help reintroduce these patterns, enhancing pollination despite declining pollinator populations.
4. Ecological networks: By improving our understanding of plant-pollinator interactions, we can better support and manage these crucial ecological relationships.
5. Plant breeding: Our work identifies genes important for creating floral patterns. This knowledge can be used in breeding programs to develop more pollinator-friendly crop varieties.
6. Conservation: For endangered plant species, understanding the genetics of floral patterns could aid in conservation efforts by ensuring plants remain attractive to their pollinators.
In essence, this research bridges fundamental biology, applied agricultural science, and ecology, offering potential benefits from improving human health to enhancing crop yields and supporting biodiversity.
Q: You’ve indicated that the next step is to explore the signals responsible for generating early petal patterns. What specific signals are you looking to identify, and how do you think they might operate across other plant species or organs?
A: At this stage, we’re casting a wide net in our search for the signals responsible for early petal patterns. It’s an exciting but challenging process because the signal could be almost anything. We initially looked at plant hormones, particularly auxin, which plays a crucial role in many aspects of plant development. While we’ve found that auxin is important for controlling growth, we don’t believe it’s the primary signal we’re looking for in pattern formation.
Interestingly, we’ve recently discovered molecules in petals that are similar to animal neurotransmitters. It’s a fascinating possibility that evolution might have repurposed these neurotransmitter-like molecules to “paint” flower patterns. We’re currently investigating this intriguing lead.
Our search isn’t limited to these possibilities, though. We’re also exploring small proteins or peptides that can move between cells, lipid molecules like sterols, and even ions such as calcium. Essentially, we’re investigating every known type of signaling molecule.
This is undoubtedly a massive undertaking, but we believe it’s worth the effort. The question of how flowers create their patterns has puzzled scientists for a long time, and we suspect that the mechanisms we uncover might be universal across plant species and even applicable to other plant organs like leaves. The potential for broad impact makes this research particularly exciting and valuable. If it were a phenomenon unique to hibiscus, it might not warrant such an extensive investigation.
However, given that we believe we’re uncovering universal principles of plant development, the effort is more than justified.
Q: What excites you most about this discovery, and what potential directions do you see your research heading in over the next few years?
A: What excites me most is the realization of how much we still have to learn, especially in plant biology. I recall a senior professor once suggesting that all the important discoveries had already been made, which I found quite disheartening at the time. However, as I’ve progressed in my research, I’ve come to understand just how wrong that perspective was. There’s an enormous amount we don’t yet understand, particularly in plants, which are comparatively understudied relative to animals.
I’m continually amazed by nature’s creativity. When we began this research, I thought I could imagine all the possible ways a bullseye pattern might form, but nature surprised us with something entirely unexpected. It’s thrilling to have the tools now to uncover these mysteries and get real answers to questions that have long puzzled scientists.
Looking ahead, I’m particularly excited about identifying the signals responsible for these patterns. I’m also keen to understand how the pigment molecules we’ve studied aren’t just passive colorants but play active roles in cell behavior. This could have implications far beyond plant biology, potentially offering insights relevant to human health.
Another intriguing direction is exploring why bullseye patterns persist in nature. Is it due to different pollinators, or are there other factors at play? These questions open up fascinating avenues for future research.
Lastly, I’m always gratified when our research reaches a wider audience. As scientists primarily engaged in fundamental research, our main product is knowledge. Sharing that knowledge is crucial; otherwise, our work loses its purpose. Seeing our discoveries spark interest and potentially lead to applications is incredibly rewarding and motivates us to continue pushing the boundaries of our understanding.
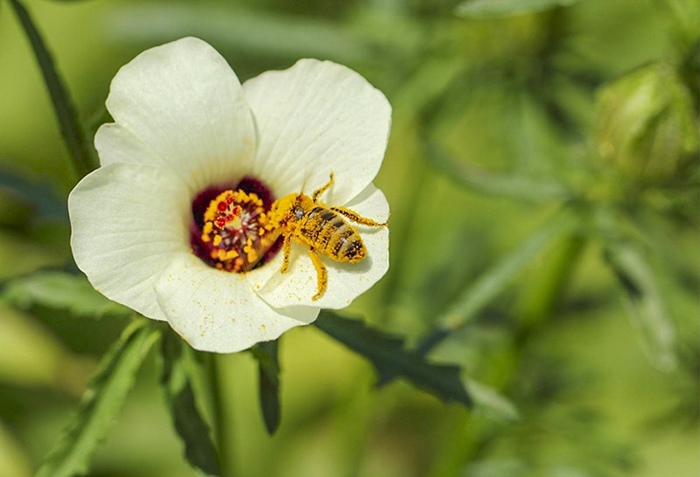
Dr. Moyroud and her team’s pioneering research into hibiscus flower pre-patterns marks a significant leap in our understanding of plant biology and evolution. By revealing the invisible blueprints guiding petal development and demonstrating bees’ preference for larger bullseyes, this study underscores the intricate dance between plants and pollinators. These findings not only illuminate the complexities of nature’s design but also have far-reaching implications for conservation, agriculture, and our broader understanding of biodiversity. As we unravel these microscopic mysteries, we gain invaluable insights that could help protect endangered species, enhance crop pollination, and deepen our appreciation of the natural world’s interconnectedness. This research serves as a powerful reminder of the endless wonders still awaiting discovery, challenging us to look beyond the surface and appreciate the hidden artistry of life.