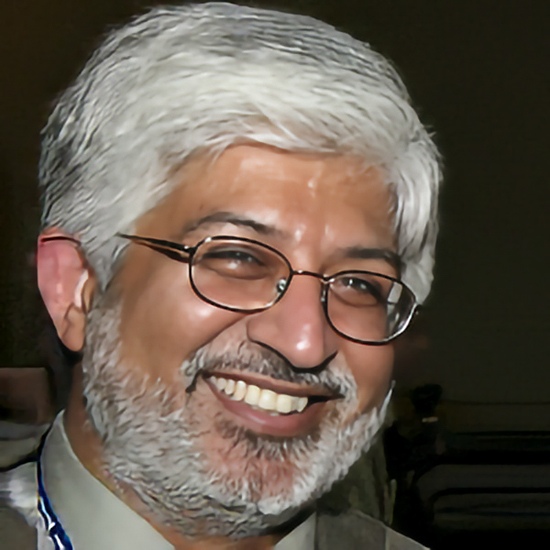
When Quantum Meets Classical: A New Era of Internet Communication
In a breakthrough that could reshape the future of telecommunications, Northwestern University researchers have achieved what many thought impossible: quantum teleportation over fiber optic cables already carrying conventional internet traffic. This achievement, led by Professor Prem Kumar, demonstrates that quantum and classical communications can coexist in our current infrastructure, potentially revolutionizing how we approach the future of secure communications.
We recently had the privilege of interviewing Professor Kumar, and the following is his detailed explanation of this complex field and the significant strides his team has made.
Classical vs. Quantum: A Tale of Two Systems
To understand the significance of this breakthrough, Professor Kumar first explains the fundamental difference between classical and quantum communication, using our everyday experiences as a starting point.
“Let’s consider classical data transmission—like what we’re doing right now, communicating across the world,” Kumar explains. “My voice is converted into electrical signals, digitized into ones and zeros, then sent through various channels like wireless signals and fiber optics. At your end, it’s converted back into audio. Essentially, we send bits—zeros and ones—from one location to another.”
Quantum transmission, however, operates on entirely different principles. Instead of simply sending bits, it involves transmitting the entire quantum state of a particle. While one could theoretically send the particle itself, quantum teleportation achieves this transfer without physically moving the original particle.
The distinction becomes clearer when comparing classical and quantum information:
“Classical information is basically a two-level system, like a coin with heads or tails, or an electrical signal that’s either high or low,” Kumar notes. “It’s always in one definite state. But quantum information can exist in what we call a superposition—meaning the particle can be in both states simultaneously.”
To illustrate this concept, Kumar offers an accessible analogy from everyday life: “In a 3D movie theater, one lens of your glasses might only let in horizontally polarized light and the other lens vertically polarized light. That’s classical separation. But in quantum scenarios, a single photon can carry both polarizations simultaneously. Communicating this entire superposition state—rather than just heads or tails—is what we call quantum communication.”
This ability to exist in multiple states simultaneously, famously illustrated by Schrödinger’s cat thought experiment, is what gives quantum systems their unique power and also makes quantum communication fundamentally different from classical data transmission. While classical communication simply transfers bits (0s and 1s), quantum communication transfers the complete quantum state, including all possible superpositions.
Breaking the Quantum Barrier
Conventional wisdom held that quantum signals—carried by single photons—would be overwhelmed by the millions of light particles used in classical data transmission. However, Kumar’s team discovered a creative solution: by carefully mapping how light scatters within optical fibers, they identified a “quiet” wavelength where quantum signals could travel undisturbed.
“We chose 1290 nanometers very carefully after measuring where the Raman scattering photons are and then created our quantum channel there,” explains Kumar. This wavelength selection proved crucial, allowing quantum signals to coexist with powerful classical communications operating at around 1550 nanometers.
The Challenge of Light Scattering
One of the primary obstacles the team faced was Raman scattering—a phenomenon where light interacts with the molecular vibrations in glass fiber. “The fiber is basically a very thin light pipe, a solid piece of glass,” Kumar explains. “When light propagates through it, it feels the vibration of the glass molecules.” These vibrations can scatter light into different wavelengths, potentially drowning out delicate quantum signals.
The team’s solution was methodical and precise. Through careful study, they identified wavelength regions where Raman scattering was minimal—similar to finding transparent windows in the atmosphere. This led them to select 1290 nanometers for their quantum channel, while classical communication operated at around 1550 nanometers.
The Role of Entanglement and Quantum Memory
Central to quantum teleportation is the concept of entanglement—what Einstein famously called “spooky action at a distance.” The process requires pre-shared entangled photon pairs between the sender (typically called Alice) and receiver (Bob). “It’s a resource,” Kumar notes, explaining that each teleportation event consumes one entangled pair.
The challenge lies in storing these entangled states. Quantum memories—devices capable of preserving quantum states—remain a critical area of research. While various approaches using atoms, ions, or solid-state systems show promise, creating efficient, long-lived quantum memories remains one of the field’s major challenges.
The Technical Achievement
The experiment successfully demonstrated quantum teleportation while the fiber simultaneously carried 400 gigabits per second of classical data—equivalent to streaming roughly 100,000 high-definition movies simultaneously. The team achieved this over a distance of 30 kilometers, using sophisticated filtering techniques to ensure the quantum signals remained intact.
“The level of interference we encountered from the Raman process was comparable to our detectors’ natural dark counts,” Kumar notes, referring to the background noise inherent in quantum detection systems. This breakthrough suggests that quantum communication could potentially utilize existing fiber optic infrastructure rather than requiring an entirely new network.
Understanding Quantum Teleportation
Unlike classical data transmission, which converts information into electrical signals and then into light pulses, quantum teleportation involves a phenomenon called entanglement. This process allows the quantum state of one particle to be instantly reflected in another particle, regardless of the distance between them.
However, Kumar emphasizes an important distinction: while the quantum state change occurs instantaneously, actually using this information still requires classical communication bounded by the speed of light. “The quantum state collapse is instantaneous but unactionable without the classical information,” he explains.
Security Implications and Real-World Applications
The implications for security are profound. Unlike classical encryption, which relies on mathematical complexity and could potentially be broken by future quantum computers, quantum communication offers security based on fundamental physical laws. “Current encryption systems are vulnerable to being recorded now and decoded later when more powerful computers become available,” Kumar explains. “Quantum communication could provide a more future-proof solution.”
Potential applications extend beyond secure communication. The technology could enable distributed quantum computing, where quantum processors in different locations are linked through quantum teleportation. This could be particularly valuable for creating larger-scale quantum computing systems without having to build massive individual processors.
From ARPANET to Quantum Networks: A Historical Parallel
Kumar draws an illuminating parallel between today’s quantum networks and the early days of classical internet. “We’re at the same stage as classical communication was in the 1970s,” he says, referring to the era when ARPANET—the internet’s predecessor—was just beginning. Just as pioneers like Vint Cerf and Bob Kahn developed protocols like TCP/IP that still form the backbone of today’s internet, researchers are now laying the groundwork for quantum communication protocols.
Looking Ahead: Challenges and Possibilities
Despite this significant advancement, Kumar acknowledges that the realization of practical quantum networks remains a long-term goal. Significant technological hurdles must still be overcome, including the development of quantum memories capable of storing entangled states, the creation of quantum repeaters to enable long-distance transmission, the implementation of robust quantum error correction protocols, and the seamless integration of these novel technologies with existing network infrastructure. Nevertheless, Kumar remains optimistic about the future, stating, “The pace of technology development is much faster now. What took 50 years in classical communications might take only 10 to 20 years in quantum communications.”
The Path Forward
The Northwestern team is already planning their next steps. They aim to move their experiment from the controlled laboratory environment to real-world underground fiber network, testing the system’s resilience in practical conditions.
This research, published in the journal Optica, represents more than just a technical achievement—it’s a paradigm shift in how we think about quantum communication infrastructure. Rather than building entirely new networks, Kumar’s work suggests we might be able to integrate quantum communications into our existing fiber optic infrastructure, significantly accelerating the pathway to practical quantum networks.
“We’re at the beginning of a new era in communications,” Kumar concludes. “The integration of quantum and classical signals in the same fiber infrastructure could revolutionize how we approach secure communication in the future.”
xxxxx
This research was conducted at Northwestern University’s Center for Photonic Communication and Computing, under the direction of Professor Prem Kumar.
Reference: Prem Kumar, Gregory S. Kanter, Fei I. Yeh, Joe J. Mambretti, Jordan M. Thomas, Scott J. Kohlert, and Jim Hao Chen. “Quantum teleportation coexisting with classical communications in optical fiber.“ Optica, 19 December 2024.