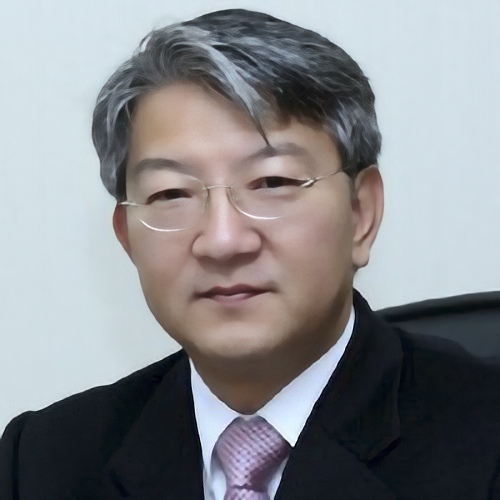
Bacteria-Powered Plastics: A Breakthrough in Thermally Stable and Biodegradable Polymers
In the quest to reduce reliance on petroleum-based plastics, bioengineers worldwide have been experimenting with plastic-producing microbes. Now, a team of researchers from the Korea Advanced Institute of Science and Technology (KAIST) has achieved a groundbreaking feat. For the first time, they have engineered bacteria capable of producing thermally stable, biodegradable plastics with properties similar to polystyrene and PET (polyethylene terephthalate).
This breakthrough, recently published in Trends in Biotechnology, represents a major leap toward sustainable alternatives to conventional plastics, particularly those used in packaging and industrial applications. The new polymer’s potential extends beyond typical uses, with researchers already exploring its applicability in the biomedical field, especially for drug delivery systems.

The Advantages of Microbial Synthesis
The researchers focused on producing phenyllactate (PhLA) through microbial synthesis, which offers several advantages over chemical synthesis. These include milder reaction conditions, reduced environmental impact, lower costs, and better development prospects. Importantly, this process utilizes renewable biomass, such as glucose, to produce biodegradable polymers.
The Challenge of Aromatic Structures
One of the biggest obstacles in bio-based plastic production has been the inclusion of ring-like “aromatic” structures in the polymers, which are essential for creating plastics with the rigidity and thermal stability required for many applications. Aromatic compounds, such as those found in polystyrene and PET, have proven toxic to most microorganisms, making it difficult to produce these polymers using bioengineering.
However, the KAIST team, led by renowned chemical and biomolecular engineer Prof. Dr. Sang Yup Lee, devised a novel approach to overcome this challenge. By constructing a unique metabolic pathway and engineering a custom polymerase enzyme, the researchers were able to enable E. coli bacteria to produce and tolerate the aromatic monomer phenyllactate.
Overcoming Key Challenges
The team faced three major challenges:
E. coli naturally lacks the metabolic machinery to produce PhLA in large quantities.
No natural polymerase was available to polymerize PhLA into poly(PhLA).
A stable storage structure within cells for the poly(PhLA) polymer was needed.
To address these issues, the researchers employed systems metabolic engineering, combining synthetic biology, systems biology, and evolutionary engineering. They enhanced metabolic flux towards PhLA, engineered a PHA polymerase enzyme based on computer simulations, and introduced heterogeneous phasin proteins to create a stable environment for the polymer.
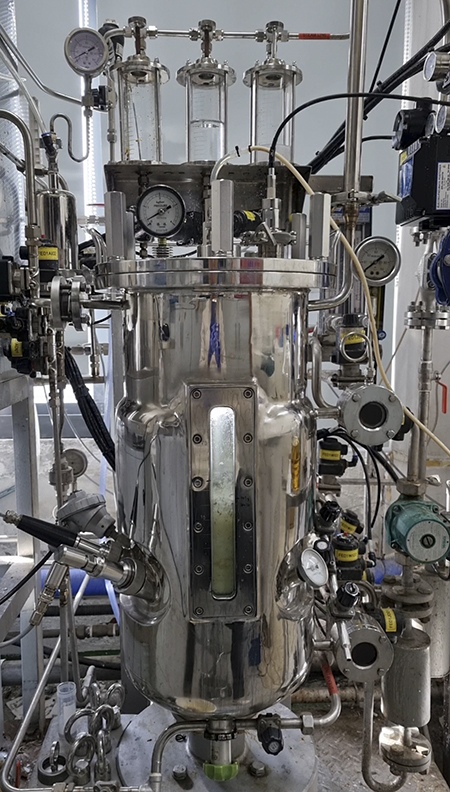
Dealing with Aromatic Ring Toxicity
The team mitigated the toxicity of aromatic rings to microbial cells by polymerizing the PhLA monomer into a polymer, reducing the concentration of toxic compounds. They also introduced phasin proteins, which surround the hydrophobic surface of the aromatic polymer, creating a stable, separated space for the polymer chains within the cells.
Breaking New Ground with Bioengineering
After refining both the metabolic pathway and enzyme, the researchers tested their new method in fermentation vats, achieving a production titer of 12.3 g/L of the polymer. While this titer is a promising start, the team aims to increase it to over 100 g/L to make large-scale commercial production viable.
Promising Applications in Medicine and Beyond
The potential applications of this new bioplastic are vast. The newly produced amorphous low-molecular weight poly(PhLA) is especially suitable for drug delivery systems due to its biodegradability and appropriate in vivo degradation rate. Its amorphous nature helps prevent unwanted crystallization of drug delivery nanoparticles, while the aromatic moieties enhance drug loading and stability.
Future Directions
The researchers are exploring ways to increase production yield through multidisciplinary approaches, including AI-driven simulations to identify bottlenecks in the metabolic machinery. They’re also working on developing other types of aromatic monomers and polymers with higher molecular weights for industrial applications.
Additionally, the team is investigating the production of various copolymers of PhLA with other monomers, which could lead to a wide range of properties and applications. This includes the potential for novel copolymers of PhLA and aliphatic monomers such as lactate, glycolate, and others.
Conclusion
This research represents an exciting step toward the production of bio-based plastics that could one day replace petroleum-derived plastics in a wide range of industries. While there is still much work to be done to optimize production and scale up the method, the future of plastic manufacturing may well lie in the hands of bioengineers like Prof. Lee and his team.
“I believe biomanufacturing will be key to addressing climate change and the global plastic crisis,” Lee emphasizes. “International collaboration will be crucial in promoting bio-based manufacturing to ensure a better future for our environment.”
xxxxx
Acknowledgements: This research was supported by the National Research Foundation of Korea, the Korean Ministry of Science, and ICT.
Published Work: For more information, see the full publication in Trends in Biotechnology: Microbial Production of an Aromatic Homo-Polyester by Lee and Kang et al.