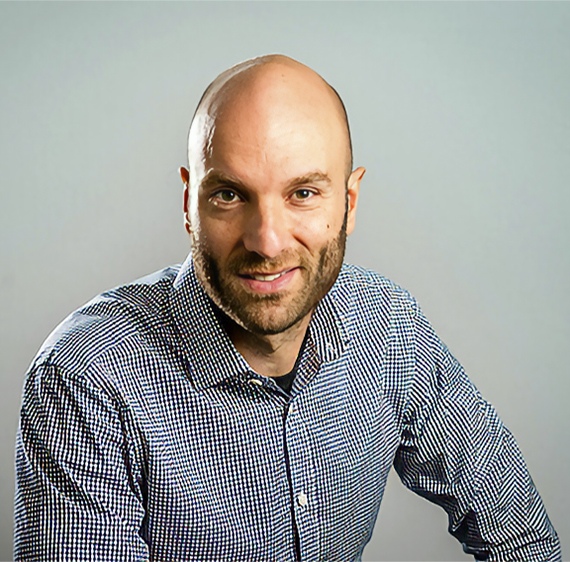
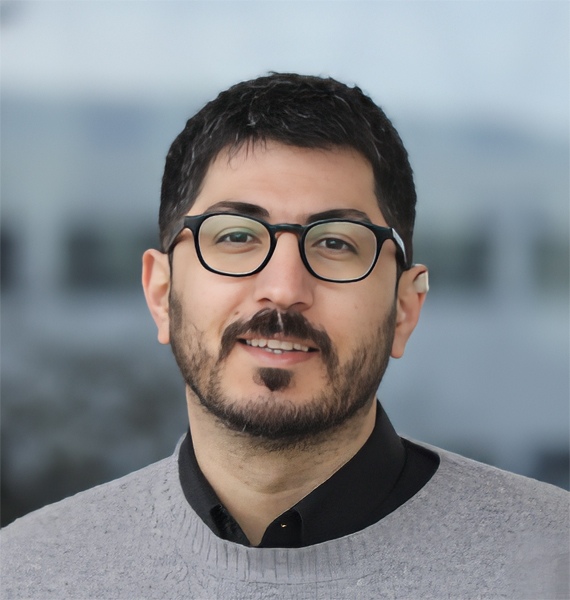
Breaking the Forever Chain: A New Hope for PFAS-Free Water
In a groundbreaking development at Oxford Brookes University, Professor Iakovos Tzanakis and Dr. Morteza Ghorbani have engineered an innovative solution to one of environmentalism’s most persistent challenges: the removal of “forever chemicals” from our water supplies. Their hydrodynamic reactor, utilizing advanced bubble cavitation technology, presents a promising path forward in the battle against toxic per- and poly-fluoroalkyl substances (PFAS) contamination. This transformative research, published in the June 28, 2024, issue of Chemical Engineering Journal, addresses the global crisis of PFAS infiltration in water sources through industrial waste, landfills, and agricultural runoff.
PFAS chemicals, present in everyday products since the 1930s—from waterproof clothing to nonstick cookware—have left an indelible mark on our environment and health. Linked to serious health conditions including cancer and liver damage, these substances have proven remarkably resistant to conventional treatment methods. However, initial testing at Sweden’s Hammarby Sjöstad wastewater treatment plant has yielded impressive results: the team’s reactor achieved a 36% degradation rate of 11 common PFAS variants in just 30 minutes, without additional chemical interventions.
Recently, we had the privilege to interview Professor Tzanakis, who specializes in Engineering Materials and Cavitation Bubble Dynamics, and Dr. Ghorbani, a Royal Society-Newton Fellow and expert at Microfluidic Devices at Oxford Brookes. These pioneering scientists shared their insights into the development of the hydrodynamic reactor and their vision for scaling up this promising solution to address one of our most pressing environmental challenges.
Q: Could you tell us about your background and current research focus?
Professor Tzanakis: I’m a Professor in Engineering Materials at Oxford Brookes University, specializing in cavitation bubble dynamics. My initial research focused on improving aluminum alloy structures and developing nanomaterials. However, I’ve now applied this expertise to wastewater treatment. My current work centers on utilizing cavitation processes to eliminate PFAS chemicals from water, representing a significant shift from my original materials engineering focus to environmental applications.
Dr. Ghorbani: In my dual roles as an Associate Professor at Sabanci University and a Royal Society-Newton Fellow at Oxford Brookes University, I focus on the physics of hydrodynamic cavitation at the microscale level. My research involves studying these processes within microfluidic devices and applying this knowledge to various environmental challenges. This includes not only PFAS degradation but also addressing other water contaminants such as bacteria, pharmaceuticals, microalgae, and microplastics. My goal is to bridge fundamental physics with practical environmental solutions.
Q: What are PFAS, and why are they a significant environmental concern?
Professor Tzanakis: PFAS, or per- and polyfluoroalkyl substances, are highly fluorinated compounds that have become a major environmental challenge. Humans have been manufacturing these chemicals since the 1950s and 60s for various products. However later scientists realized a critical problem: we can create them, but we can’t break them down. This persistence is due to the extremely strong bond between carbon and fluorine atoms in their structure. They’re called “forever chemicals” because they can persist in the environment for centuries; nature lacks the mechanisms to decompose them.
Dr. Ghorbani: Currently, there are more than 10,000 different PFAS compounds, commonly found in fluorochemical industries and firefighting equipment. These chemicals are particularly concerning due to their ubiquitous presence in everyday items—from food packaging like pizza boxes and sandwich bags to non-stick cookware. The real environmental challenge emerges at the end of these products’ lifecycle. When disposed of in landfills, these chemicals can leach into groundwater systems, eventually contaminating our drinking water sources. It creates a vicious cycle of contamination that needs to be interrupted.
Professor Tzanakis: What’s alarming is how this contamination cycle perpetuates itself. PFAS enter our water systems through various pathways—industrial discharge, landfill leachate, and everyday product disposal. This is why we’re focusing our research on developing radical solutions to break this cycle through our new promising bubble technology.
Q: Can you explain how bubble cavitation works to break down PFAS compounds?
Dr. Ghorbani: Cavitation bubbles are fundamentally different from the bubbles we see in boiling water. When cavitation bubbles form and subsequently collapse, they release an enormous amount of energy. This energy, combined with various flow kinetics in our reactor, appears to be key in targeting and degrading PFAS compounds.
The process is quite complex, and we’re still investigating the exact mechanisms at play. These collapsing bubbles release different types of energy—both mechanical and chemical effects—and we’re studying which specific effects are most responsible for PFAS degradation. While we continue to research the precise mechanisms, we know that the combination of bubble energy, flow kinetics, and other parameters in the working fluid creates conditions capable of breaking down these traditionally persistent chemicals.
Professor Tzanakis: What’s exciting is that our method harnesses natural physical processes rather than requiring additional chemical treatments. However, understanding the exact mechanisms will be crucial for optimizing the technology’s effectiveness and scaling it up for broader applications.
Q: Could you explain the design factors of your hydrodynamic reactor and its technical specifications?
Professor Tzanakis: Our reactors are designed with adaptable working principles enabling significant flexibility and optimization. While the core concept remains the same, we can adjust various parameters such as the surface characteristics of materials, liquid kinetics, and pressure variations within our network of pipes and fittings to precisely control the generation and behaviour of cavitation bubbles. To put this into perspective, our system operates at speeds to about 40–50 meters per second—approximately 150 kilometers per hour. Imagine balloons (representing bubbles) forming in rushing air (representing wastewater): the wastewater moves at high speed, creating bubbles due to pressure differences. When these bubbles collapse, they release intense effects that contribute to PFAS degradation.
Dr. Ghorbani: The bubble collapse generates enormous energy that manifests in various ways—mechanical effects, chemical effects, flow patterns, shockwaves, and free radicals. Our focus has been particularly on flow patterns, as these provide crucial insights for designing and configuring our microfluidic devices. By understanding and controlling these flow patterns, we can better tune the shockwave and chemical aspects of the process. This understanding allows us to optimize both the surface modification and the overall performance of PFAS removal, offering a powerful and efficient solution.
Q: How does your reactor compare to conventional PFAS treatment methods?
Dr. Ghorbani: Currently, there’s no standardized industrial-scale technology available for PFAS removal in treatment plants. While some pilot setups exist, our technology stands out for two key reasons. First, we don’t use any chemicals—we rely solely on bubble energy released. Second, we don’t require external energy sources like electricity, as we harvest the energy already available in wastewater treatment plant tubing systems. These features make our technology particularly novel and superior to existing methods. Importantly, this is one of the rare technologies that could be feasibly scaled up, as other methods often face prohibitive costs and energy requirements when attempting to scale.
Professor Tzanakis: The technology’s versatility is particularly exciting—it can be both scaled up and scaled down effectively. The system’s simplicity, based on a network of pipes with some modifications, makes it highly adaptable. Since it’s electricity-free and only requires pressurized gas to generate pressure differences, it has potential applications in rural areas or developing countries without reliable electricity access. People could potentially purify well water in situ at home, making it a truly versatile solution.
Q: What were your findings from testing at the Hammarby Sjöstad plant?
Professor Tzanakis: We tested the reactor against the 11 most common PFAS types at very low concentrations, ranging from 5 to 10 nanograms per liter. To put this in perspective, the U.S. Environmental Protection Agency sets drinking water limits at 4 nanograms per liter. Within just 30 minutes of treatment, we managed to reduce PFAS levels in all cases to below this threshold, and in some cases, even further (below 1 nanogram per liter). Overall, we achieved an average degradation rate of 36%, which is quite promising for this technology.
Dr. Ghorbani: Two important findings stand out from these tests. First, our technology successfully removed all types of PFAS components—both long-chain and short-chain variants. This is significant because most existing technologies only work effectively on certain types. Second, we demonstrated success at extremely low concentrations—around 1 to 2 nanograms per liter—achieving reduction rates of 40–50% in some cases. This effectiveness at such low concentrations is particularly noteworthy for practical applications.
Q: What are the main challenges in scaling up this technology?
Professor Tzanakis: The challenges vary depending on whether we’re scaling up or down. When scaling down, clogging becomes a major concern as smaller channels are more susceptible to blockages. For scaling up, erosion is the primary challenge—the continuous collapse of bubbles creates shockwaves that can eventually damage the reactor surfaces over time. This means we need to focus on materials technology to identify the most durable and suitable materials for long-term operation.
Dr. Ghorbani: Beyond technical challenges, one of our biggest hurdles is gaining industry acceptance. Wastewater treatment plants typically prefer to use conventional technologies, like ozonation or other advanced oxidation processes, despite their high energy consumption and cost inefficiency. Convincing them to adopt our novel approach requires demonstrating its value conclusively. However, our reactor has proven highly adaptable—it’s compatible with volumes ranging from very small up to 20 liters with next step being 200 liters, making technical scaling relatively straightforward.
Professor Tzanakis: There’s also an economic angle to consider. In recent discussions with UK water companies, I learned that many are struggling with the high energy costs of current UV treatment methods for water disinfection. They’re actively seeking new, more energy-efficient technologies. This presents an opportunity for our system, which operates with significantly lower energy requirements and could potentially replace these costly, energy-intensive technologies.
Q: What role did international partnerships play in the development of this technology?
Professor Tzanakis: International partnerships have been essential to our success, bringing diverse perspectives to a common challenge. Each partner contributes unique strengths and resources, creating a synergy that wouldn’t be possible working in isolation. For instance, different countries brought different expertise and capabilities to the project, and this combination proved crucial in achieving our results.
The collaborative nature of our work exemplifies how complex environmental challenges require global solutions. Our partnerships across multiple countries and institutions allowed us to pool resources, share knowledge, and accelerate development in ways that would have been impossible for any single team working alone. The success of our reactor is a testament to the power of international scientific collaboration.
Q: How does your technology align with global initiatives to reduce PFAS contamination?
Dr. Ghorbani: Our approach is strongly aligned with European Commission guidelines and directives. Currently, there’s a push to encourage wastewater treatment plants to upgrade their facilities for PFAS removal. We anticipate these recommendations will soon become mandatory requirements. It’s crucial to begin implementing and testing new technologies now, as the assessment and integration process can take several years. We can’t afford to wait until regulations become stricter to start testing solutions—the time to act is now.
Professor Tzanakis: We’re facing a legacy problem that spans over 70 years. While current initiatives are rightly focusing on reducing new PFAS production, we still need to address the billions of existing PFAS-containing products and the trillions of PFAS molecules already in our environment. Even with immediate solutions, cleaning our world of these “forever chemicals” will take years. This makes our technology particularly relevant as it offers a practical approach to addressing this enormous environmental challenge.
Q: What’s your timeline for full-scale implementation, and how has recent recognition influenced your work?
Professor Tzanakis: We’re optimistic about demonstrating our technology’s effectiveness at treating substantial volumes—up to 200 liters—within the next couple of years. Once we achieve this milestone, scaling up to industrial levels should be feasible. The recent global media attention our research has received has been invaluable, as this recognition will likely help secure additional funding to advance our work toward full implementation.
Dr. Ghorbani: Implementation requires a comprehensive approach. While we can move quickly with the right funding and international consortiums, proper monitoring systems must be developed alongside the technology. We’re already establishing connections for implementation in Turkey, Sweden, and the UK, but we’re eager to expand globally. Importantly, our technology shows promise beyond just PFAS removal—we’re seeing success with other micropollutants, bacteria, and active pharmaceutical ingredients. We’re even exploring applications in household appliances, such as washing machines, where the technology could enable water reuse.
Looking ahead, we envision developing standardized protocols for different contaminants, though each target may require specific tuning. While a universal “one recipe” solution might be possible in the future, the encouraging news is that our technology has already demonstrated effectiveness against PFAS and a broad spectrum of other contaminants simultaneously. This versatility, combined with growing recognition and support, positions us well for broader implementation.
Conclusion
As global awareness of PFAS contamination grows and regulations tighten, the Oxford Brookes team’s innovation offers a promising solution to a decades-old environmental challenge. Their chemical-free, energy-efficient approach could revolutionize water treatment worldwide, providing a practical tool for environmental cleanup efforts that will span generations. With continued development and implementation, this technology may finally offer a way to break the endless cycle of PFAS contamination, bringing us closer to a future of cleaner, safer water for all.
Websites:
https://cav-it.co.uk/
https://www.brookes.ac.uk/profiles/staff/iakovos-tzanakis