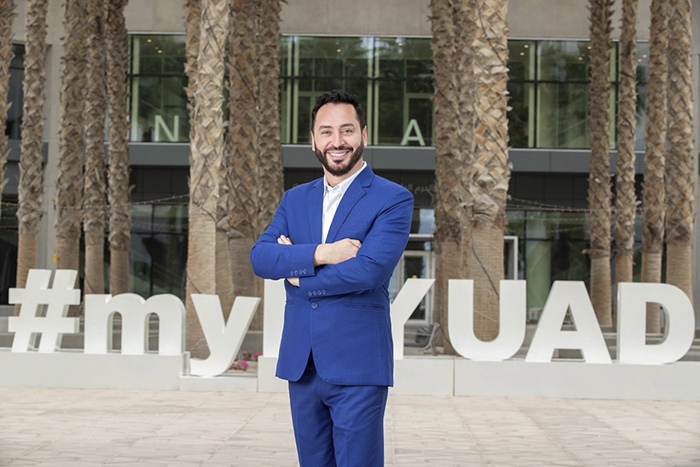
Molecular Regeneration: Inside Dr. Panče Naumov's Groundbreaking Research on Self-Healing Crystals
Crystals, traditionally seen as symbols of rigidity and brittleness, are now revealing a remarkable hidden characteristic: the ability to heal themselves. Leading this breakthrough is Dr. Panče Naumov and his research team at New York University and New York University Abu Dhabi. Since the mid-2010s, they have been transforming the field of materials science by uncovering and explaining the self-repairing behavior of molecular crystals. Their work offers profound insights into the internal dynamics of solid materials and paves the way for a new generation of durable, sustainable, and adaptable technologies. This groundbreaking research was featured in a detailed article published in Nature Reviews Chemistry on April 14, 2025, marking a major advance in the study of responsive materials.
A Conceptual Leap: Crystals as Soft Matter
The renewed interest in self-healing molecular crystals, which began to gain momentum around 2016, was driven by a pivotal shift in thinking. “Around 2015, we realized that organic crystals could be classified as soft materials, similar to plastics and rubber, but with the added feature of structural order, which makes them quite unique,” explains Dr. Naumov. This realization led to a key hypothesis: if conventional soft materials can heal themselves, perhaps highly ordered crystalline materials can do the same.
Inspired by dynamic covalent chemistry used in self-repairing polymers, where chemical bonds can break and reform at room temperature without external energy, Dr. Naumov’s team set out to design organic crystals with built-in repair mechanisms. “My senior researcher, Dr. Patrick Commins, focused on synthesizing organic crystals containing disulfide bonds that could break and reform at room temperature,” Naumov recalls. While the synthesis was relatively straightforward, the challenge was proving that the healing actually occurred.
Navigating the Crystal Maze: Challenges in Testing
Unlike polymers or gels that can be molded into standard shapes for mechanical testing, organic crystals form in specific geometries determined by their internal structure and growth conditions. Their small size and irregular shapes made traditional testing techniques impractical. “We realized we had to create new methods to test them,” says Naumov.
His team developed an innovative approach: intentionally damaging a crystal, mounting it on a miniature tensile tester capable of detecting very small forces, breaking it completely, then carefully aligning and rejoining the fragments at the break point. After allowing the crystal to heal for 24 hours, they measured the force required to break it again and compared it to an untouched crystal. “We described these protocols in our review paper to help other researchers build on this work,” Naumov adds, stressing the need for standardized methods in this emerging field.
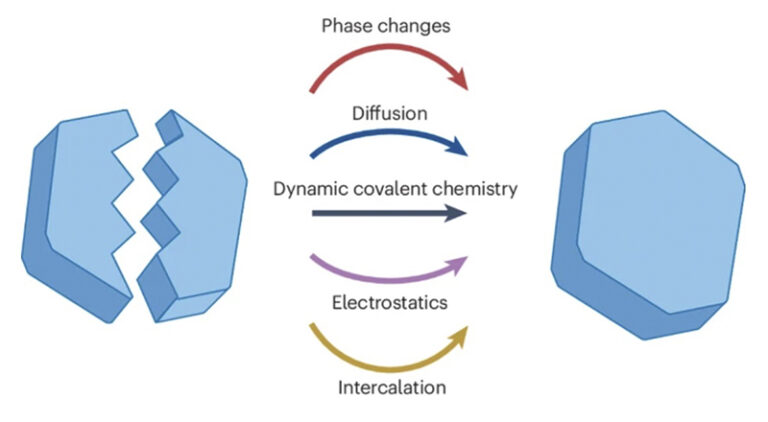
Mechanisms of Mending and the Advantage of Order
Since their first report nearly ten years ago, Dr. Naumov’s group and others have identified a wide range of mechanisms by which molecular crystals can heal. These include dynamic covalent bonding, phase transitions, electrostatic interactions, intercalation, remote-triggered repair, and possibly pericyclic reactions. Early results showed limited healing efficiency, with crystals recovering around 7 to 8 percent of their strength, leading to skepticism when compared to polymers that can fully recover. “The initial low efficiency made some in the scientific community hesitant,” Naumov acknowledges. However, consistent findings across multiple groups, published in leading journals such as Science and Nature, have since confirmed that self-healing is indeed a real property of some crystals.
Interestingly, evidence of this behavior exists in earlier research. During the Cold War, U.S. national laboratories observed self-healing in inorganic crystals such as sodium chloride for nuclear storage applications, but these findings were not widely circulated.
What sets molecular crystals apart from materials like polymers is their long-range structural order. “Polymers are inherently disordered. While we know they can heal themselves, it is difficult to determine exactly how,” Naumov explains. “In crystals, healing usually occurs at the break, while the rest of the material remains unchanged.” This order allows scientists to study the structure using techniques like X-ray diffraction and surface-sensitive methods. It also gives rise to anisotropic properties—meaning the material behaves differently depending on direction—which is not the case for isotropic polymers. This structural order is key to many future applications.
From Laboratory to Longer Lifespan: Potential Applications
According to Naumov, the goal is to harness the unique combination of structure, flexibility, and self-repair in organic crystals to replace rigid silicon-based components in electronics and optics. “People once argued that organic crystals were unsuitable because they wore down too easily,” he says. “Now that we know they can heal, the situation has changed.”
Imagine smartphones or wearable technology built with crystal components that can repair themselves after damage. Such materials could significantly extend the lifespan of devices and reduce electronic waste. This idea has drawn interest from large technology companies and national research organizations.
The environmental benefits are equally important. Organic crystals are composed of lightweight elements such as carbon, hydrogen, nitrogen, oxygen, and sulfur, and do not contain toxic heavy metals often found in inorganic materials. “These crystals can potentially break down completely,” Naumov explains, pointing to future uses such as biodegradable optical waveguides for biomedical applications like photodynamic therapy, where biocompatibility is essential.
The Challenge of Shaping and Triggering the Healing Process
Despite the exciting potential, one major challenge remains: shaping crystals into useful forms. Unlike polymers, crystals cannot easily be molded into complex designs. “When we speak with engineers, their first concern is always about size and shape, which affects how these materials can be integrated into devices,” Naumov says. Although researchers are working on controlling crystal growth and developing post-processing methods such as cutting with light, there is currently no universal solution. “Each crystal behaves differently,” making it difficult to generalize results.
Self-healing in crystals usually requires external triggers like heat or light because these are easy to apply in laboratory settings. However, researchers are expanding the list of possible triggers. Naumov’s team has tested circularly polarized light for security applications and temperature-sensitive light emission for sensors. By combining crystals with other materials like polymers or photothermal substances such as MXenes, the range of responses can include magnetic fields, electric fields, moisture, and even infrared light. This approach also retains the advantage of growing crystals at room temperature, which saves energy compared to the high temperatures needed for inorganic materials.
Confirming the Healing and Looking Ahead
To truly confirm healing, researchers must go beyond visual evidence. While optical, electron, and fluorescence microscopy provide initial insights, more precise tools are needed. “We encourage using multiple techniques,” Naumov says. Tensile testing is one method, but more advanced imaging technologies like two-photon microscopy and computed tomography are now being used to examine the interface where healing occurs.
Looking to the future, Naumov believes the field is shifting from academic curiosity to real-world development. “We are seeing progress in several key areas,” he notes, including better testing methods and experiments focused on how repeated healing affects device longevity. Interest from engineers and organizations such as the U.S. Department of Defense suggests that the potential of these materials is beginning to be recognized.
Though challenges in manufacturing and scalability remain, the ability of some crystals to repair themselves is a game-changing discovery. It transforms how we view these materials and points to a future where devices combine unique directional properties with the power to regenerate, making them more durable and environmentally responsible than ever before.