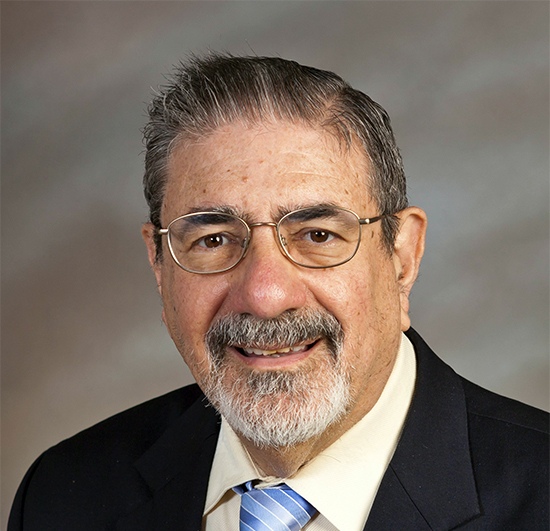
Revolutionizing Chemistry: A New Frontier in Sustainable Innovation
The air we breathe is becoming a cornerstone of revolutionary advancements, promising to reshape not only agriculture but also the broader field of chemistry. Researchers from Stanford University and King Fahd University of Petroleum and Minerals have developed a groundbreaking device that uses wind energy to extract nitrogen from the atmosphere and convert it into ammonia. This innovation has the potential to replace the century-old, energy-intensive Haber-Bosch method, creating a new benchmark for sustainable practices. The study, published December 13 in Science Advances, marks a significant milestone in sustainable chemistry.
Traditionally, the production of ammonia, a key ingredient in fertilizers, requires combining nitrogen and hydrogen under extreme pressures and temperatures. This process consumes about 2% of global energy and contributes 1.8% of annual carbon dioxide emissions. In contrast, the new method operates at room temperature and atmospheric pressure, eliminating reliance on fossil fuels. The result is a portable, scalable solution that could allow farmers to produce ammonia directly on-site, dramatically reducing costs and environmental impact.
We had the privilege of speaking with Dr. Richard Zare, senior author of this groundbreaking study, to delve deeper into the inspiration, challenges, and broader implications of this technology. The following Q&A highlights the transformative potential of this innovation.
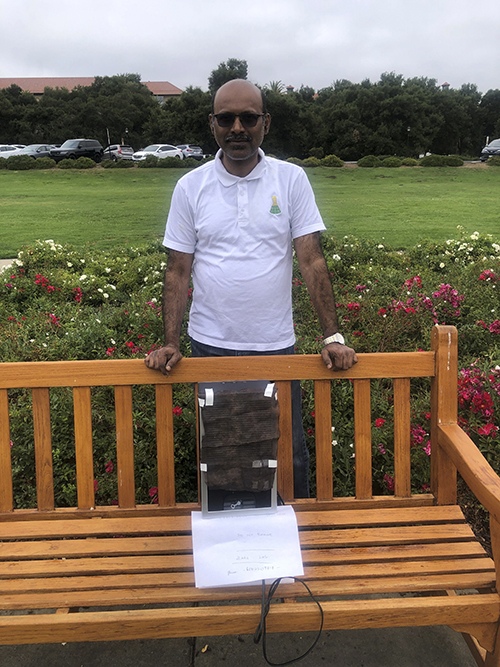
Breaking Down the Chemistry
Q: What unique chemical or physical principles enable this device to harness atmospheric nitrogen and convert it into fertilizer-grade compounds?
A: Nitrogen molecules (N2) are incredibly stable due to their strong triple bonds, making them difficult to break apart and convert into plant-usable forms like ammonia or nitrates. Traditionally, this transformation has relied on the Haber-Bosch process, an energy-intensive industrial method that operates under extreme temperatures and pressures.
This new device leverages interfacial chemistry, using the unique properties of water droplets interacting with a magnetite-coated (Fe3O4) screen to facilitate nitrogen conversion. Incomplete solvation of H+ and OH- ions at the droplet’s surface creates reactive radicals, such as hydroxyl (OH) and atomic hydrogen (H), which initiate the transformation of atmospheric nitrogen into ammonia. The process occurs at room temperature and standard pressure, requiring no external voltage or high temperatures. Driven by wind or air blowers, this green, energy-efficient technology offers a scalable alternative to traditional methods, paving the way for sustainable agriculture.
The key lies in using a magnetite-coated (Fe3O4) screen as a catalyst. By passing humid air over the screen, the gas-water interface facilitates the reactions at room temperature and standard pressure. Unlike traditional methods, this process requires no external voltage or high temperatures, making it green, energy-efficient, and scalable—a game-changer for sustainable agriculture when it can be successfully scaled.
Unanticipated Discoveries
Q: Did any unexpected scientific phenomena emerge during development that reshaped the team’s original approach?
A: Yes, we uncovered fascinating potential within interfacial chemistry that could extend beyond ammonia production. During our research, we discovered that this approach might enable the synthesis of a wide range of valuable compounds. For instance, we demonstrated the ability to transform methane (CH4), a potent greenhouse gas, into methanol (CH3OH), a liquid that is much easier to store, transport, and utilize. This finding highlights the versatility of interfacial chemistry in addressing environmental challenges.
Moreover, we explored the addition of carbon dioxide (CO2) into the reaction environment, successfully producing urea (NH2CONH2), another key fertilizer. These unexpected results have opened the door to broader applications of microdroplet chemistry, suggesting it could revolutionize the production of critical chemicals in a more sustainable and scalable way. The ongoing challenge now lies in refining and scaling these processes to harness their full potential for both agricultural and industrial applications.
Revolutionizing Fertilizer Production: From Factory to Field
Q: What are the transportation advantages of ammonia?
A: Ammonia serves as an excellent hydrogen carrier, offering significant transportation benefits in the transition toward a hydrogen economy. While hydrogen storage and transport present major challenges, converting it to ammonia creates a practical solution for easier handling.
However, current ammonia production through the Haber-Bosch process carries substantial environmental costs. Though vital for industrial and agricultural development, this energy-intensive method consumes about 2% of global energy and generates roughly 1.8% of worldwide carbon dioxide emissions, largely due to hydrogen extraction from natural gas through steam reforming. To fully realize ammonia’s benefits, developing sustainable production methods is crucial.
Q: How might this innovation transform traditional fertilizer manufacturing?
A: This innovation could fundamentally reshape fertilizer manufacturing by providing a more sustainable, cost-effective alternative to centralized production. Rather than relying on massive industrial facilities that transport ammonia to farms, this technology enables in situ production directly in agricultural fields-potentially revolutionizing the entire supply chain.
This technology opens exciting possibilities, such as integrated irrigation-fertilization systems where sprinklers equipped with metal oxide surfaces could simultaneously water and fertilize crops by generating ammonia from air and water. While currently producing smaller quantities better suited for controlled environments like hydroponic greenhouses, continued development through engineering collaboration could lead to broader applications. Success would mark a paradigm shift toward decentralized, sustainable fertilizer production integrated into daily farming practices.
Powering the Future: Sustainable Energy for Next-Generation Fertilizer Production
Q: What energy requirements are needed to deploy this technology, particularly in remote locations?
A: While this technology follows energy conservation principles, it demands significantly less power than traditional ammonia production methods. The main energy needs come from creating water droplets and moving air past the catalytic surface, rather than directly powering chemical reactions. The system can run on various power sources-batteries, wind, or standard electricity—making it highly adaptable for field use. This versatility makes it particularly suitable for farmers in remote areas without access to centralized energy infrastructure. Future developments could incorporate renewable energy sources to further enhance sustainability and deployment potential.
Q: How viable are renewable energy sources for scaling this technology?
A: Renewable energy sources like solar and wind power could effectively drive this device, creating a fully sustainable process. Solar panels could generate the necessary electricity for operating blowers and creating water droplets, particularly in greenhouse settings where the technology would be both eco-friendly and self-sufficient. The implementation path likely involves phases, progressing from current laboratory demonstrations and small-scale applications (like those at Stanford and local water sources) to larger prototypescapable of significant ammonia production. This scaling process requires both engineering advances and financial investment.
The final step toward widespread adoption involves commercialization-refining and marketing systems that integrate seamlessly into agricultural operations. When powered by renewable energy, this technology could enable farmers in remote or resource-limited areas to sustainably produce their own fertilizer, potentially revolutionizing global agricultural practices.
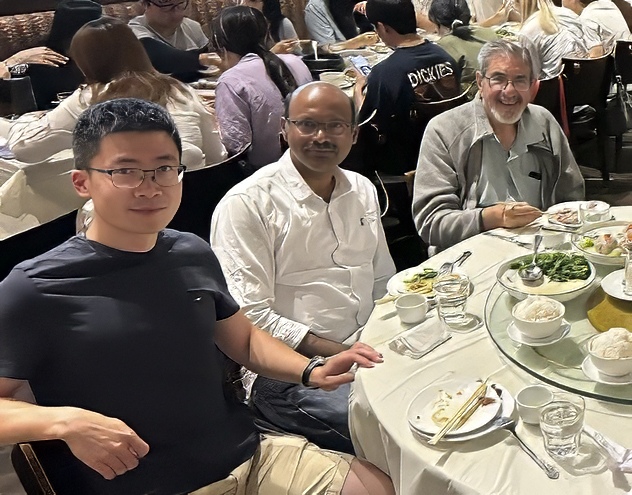
From Lab to Market: Building Global Partnerships for Sustainable Innovation
Q: What are your immediate next steps for this technology?
A: Scaling up this technology is our primary focus, and we’re making significant progress through active discussions with several startups interested in advancing this innovation. These collaborations are crucial for transforming our laboratory success into a practical, large-scale solution. The project has benefited from strong international cooperation. Dr. Xiaowei Song from China has been instrumental in my research group, while Professor Chanbasha Basheer from King Fahd University of Petroleum and Minerals in Saudi Arabia contributes valuable expertise during his summer visits.
Q: Are you exploring applications beyond ammonia production?
A: Yes, we’re investigating several exciting possibilities. For instance, our collaboration with Professor Gnanamani from IIT Roorkee in India explores using this method for pharmaceutical synthesis. Since pharmaceutical applications often require only small quantities of ammonia-milligrams or grams-they present an ideal early application for our technology. The versatility of our approach positions it as a potential game-changer across multiple industries.
Making Innovation Accessible: From Farm Fields to Global Markets
Q: How could this device benefit farmers in developing regions?
A: The device has the potential to significantly lower costs, making it an affordable solution for farmers, particularly in developing countries. By reducing production expenses, it could contribute to cheaper food, benefiting broader populations. However, determining the exact pricing will depend on various market factors outside my scientific expertise.
Q: What additional benefits might this technology offer?
A: Interestingly, the same technology produces hydroxyl radicals (OH), which can combine to form hydrogen peroxide (H2O2). This byproduct has exciting potential as a disinfectant, opening additional applications beyond agriculture. While our small group can’t explore every possibility, this technology invites collaboration and innovation, offering opportunities for others to build on our work and expand its impact across multiple industries.
Sustainable Solutions: Harnessing Water for a Greener Future
Q: What ecological effects might widespread adoption of this device have?
A: The primary positive impact would be significantly reducing energy consumption. By using water as the primary source of hydrogen for chemical reactions, this technology promotes green chemistry and leverages an abundant resource. With 80% of the planet covered in water, including saltwater which we’ve successfully tested, this approach holds tremendous potential for sustainable practices while reducing pressure on freshwater supplies. While the potential ecological benefits are vast, from sustainable farming to new applications in green chemistry, careful implementation and monitoring will be essential to ensure positive impact while mitigating any unforeseen consequences.
Expanding Frontiers: From Nanoparticles to Climate Solutions
Q: Could this technology be adapted for broader chemical production?
A: Absolutely. In our laboratory, we’ve already demonstrated broader applications, such as producing silver nanoparticles. By dissolving silver nitrate (AgNO3) in water and spraying it, we can trigger the formation of silver atoms that aggregate into nanoparticles—useful for antimicrobial applications like wound care. We’ve also successfully produced gold nanoparticles, which have applications in catalysis and advanced materials research. The unique reactivity at the gas-water interface nsuggests this technology could revolutionize the production of various industrial reagents and chemicals, expanding its impact far beyond agriculture.
Q: What types of partnerships would be most valuable in advancing this technology?
A: This invention is inherently interdisciplinary, combining chemistry, chemical engineering, and physics in its core development. We’re foreseeing exciting potential in medical applications, such as producing antimicrobial agents and therapeutic compounds. This technology also intersects with the fields of geology and climate science; we’ve discovered it can transform organic sulfur compounds into sulfates, which play crucial roles in cloud formation and Earth’s climate regulation. To fully realize this technology’s potential, we need partnerships across medical research, climate science, engineering, and policy sectors. Such collaborations could drive breakthroughs from sustainable agriculture to environmental protection, making this a truly revolutionary advancement.
Future Horizons: Transforming Chemistry for a Sustainable World
Q: How might this technology shape our future in the next 10-15 years?
A: I see it as having the potential to fundamentally reshape chemistry itself. This technology represents a significant innovation, but its true impact depends on whether it can be scaled effectively. While publishing papers is important in the academic world, the ultimate goal is to translate this research into practical applications that can transform everyday life on a global scale. If successful, it could redefine how we approach sustainable chemistry and create solutions that extend far beyond its initial scope.
Conclusion
This groundbreaking innovation has the potential to revolutionize chemistry, agriculture, and beyond. By decentralizing fertilizer production, leveraging renewable energy, and enabling green chemical synthesis, it addresses critical environmental and economic challenges.
As Dr. Zare and his team refine and improve their invention, the possibilities seem limitless—from empowering farmers in developing countries to advancing pharmaceutical and industrial applications. With interdisciplinary collaboration and global support, this technology could lead the way to a more sustainable and equitable future, redefining the limits of modern science and industry.