The video above was created by the Allen Institute.
The Microcosm Within: Inside the Groundbreaking MICrONS Brain Mapping Project
In the vast landscape of scientific discovery, while astronomers chart distant galaxies and physicists delve into the mysteries of subatomic particles, the human brain continues to be one of the most elusive frontiers. Its intricate neural circuits, which form the foundation of thought and emotion, have long defied complete mapping. However, on April 9, 2025, years of dedicated work under the Machine Intelligence from Cortical Networks (MICrONS) Project, which began around 2016, led to a groundbreaking achievement. Researchers unveiled the most comprehensive wiring diagram ever produced that integrates both the structure and function of a mammalian brain. From a fragment of mouse brain no larger than a grain of sand, scientists reconstructed the connections and recorded the activity of more than 200,000 cells in extraordinary detail. This work provides an unprecedented window into the neural architecture that supports cognition, offering a foundational model for understanding the human mind and the biological roots of neurological disorders.
Conquering the 'Impossible'
The importance of this achievement resonates deeply within the field of neuroscience. In 1979, Nobel laureate Francis Crick famously declared that mapping the precise wiring and activity within even a single cubic millimeter of brain tissue was an impossible task. Nearly fifty years later, through sustained innovation and collaboration, the MICrONS project has turned that impossibility into a transformative reality. A global consortium of more than 150 researchers from leading institutions—including the Allen Institute, Baylor College of Medicine, Princeton University, Harvard University, and Stanford University—has constructed a digital neural map of remarkable scale and detail. Data collection for this effort began around 2019 and 2020, resulting in a dataset that includes 200,000 cells, 5.4 kilometers of axonal pathways, and 523 million synaptic connections through which neurons exchange information. This vast dataset, totaling 1.6 petabytes or 1.6 million gigabytes, is now freely available through the MICrONS Explorer portal. It offers an invaluable resource to scientists across the globe, including those contributing to major research efforts such as the China Brain Project.
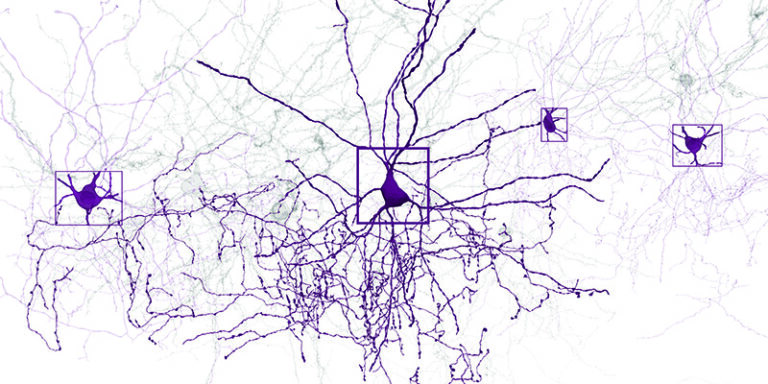
A Multi-Stage Endeavor: Integrating Function and Form
Creating this intricate map required a carefully coordinated, multi-stage process designed to capture both neuronal activity and physical connections within the same cubic millimeter of brain tissue, located at the boundary between the primary visual cortex and higher visual areas.
The process began at Baylor College of Medicine, where the team led by Andreas Tolias recorded the activity of 76,000 neurons in a living mouse as it observed a variety of visual stimuli. These ranged from complex natural movies such as The Matrix to simpler, more controlled displays like moving lines and discs. Rather than using traditional electrodes, the researchers employed calcium-sensitive fluorescence microscopy. The neurons had been genetically modified to express fluorescent molecules that emit brighter light when calcium enters the cell during an electrical spike. This allowed scientists to visually detect bursts of activity, creating an optical measure that corresponded to the brain’s electrical signals. By training machine learning models on the neuronal responses to these naturalistic visual inputs, the team was able to construct predictive digital replicas of how individual neurons would respond to a wide range of stimuli.
The second major phase followed after the mouse was euthanized and its brain tissue preserved. At the Allen Institute, the small fragment of brain was treated with heavy metal stains that infiltrated all cellular components, including membranes, organelles, and proteins. This process revealed the brain’s complete internal structure at the microscopic level. The tissue was then sectioned into more than 25,000 ultra-thin slices, each just 40 nanometers thick. Using a fleet of electron microscopes, the team captured nearly 100 million high-resolution images of these layers.
The final stage was the reconstruction. In close collaboration with Sebastian Seung’s group at Princeton University, researchers aligned the millions of two-dimensional images into a coherent three-dimensional volume. They used advanced artificial intelligence systems to trace the complex paths of individual neurons through this densely packed network. The task has been likened to navigating a massive and intricate coloring book, identifying not only the paths of the neurons but also the exact points at which one neuron communicates with another.
A particularly groundbreaking aspect of this project was the successful linking of the functional data from the living brain with the structural data from the preserved tissue. This was made possible through a process known as coregistration, which relied on using shared visual landmarks such as blood vessels and the precise locations of cell bodies. With this approach, the researchers were able to accurately map activity patterns onto the physical architecture of individual neurons.
The result is a living portrait of neural circuitry in action, combining structure and function in unprecedented detail.
“Inside that tiny speck is a forest of incredible complexity,” says Dr. Clay Reid, senior investigator at the Allen Institute and a pioneer in electron microscopy based connectomics. “It is a place to test long-standing theories and to discover truths we have never seen before.”
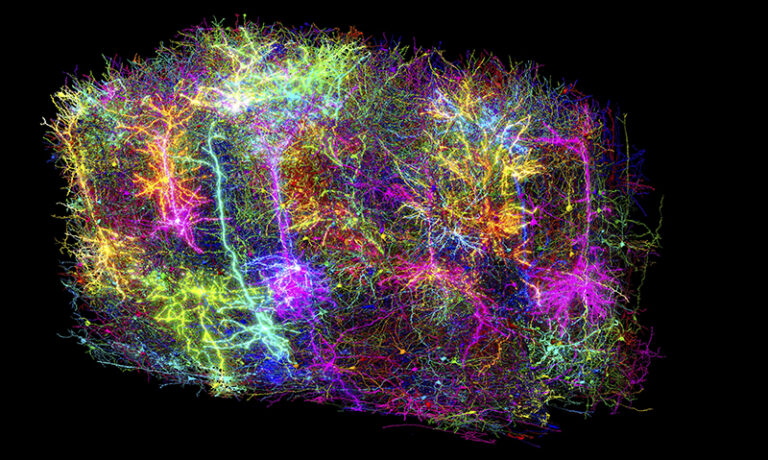
Rewriting the Neural Rulebook
The first results from the MICrONS project, published in ten separate studies across the Nature family of journals, are already reshaping long-standing ideas about how the brain is organized. One particularly striking discovery reveals the unexpectedly sophisticated role of inhibitory neurons. These cells were once thought to serve only as suppressors of neural activity, but the new data shows that they engage in a much more dynamic role. Rather than simply dampening signals, they orchestrate a delicate balance between inhibition and excitation, selectively targeting excitatory neurons to manage communication across neural networks. This refined view of inhibitory function, combined with the discovery of previously unrecognized cell types and organizational principles made possible by the dataset’s extraordinary level of detail, is helping to deepen the classification of the brain’s fundamental components.
The dataset also draws attention to key differences between species. In contrast to the highly ordered spatial features seen in the primate visual cortex—such as orientation columns and color-specific clusters—the mouse visual cortex exhibits what scientists describe as a salt and pepper pattern. In this arrangement, functionally distinct neurons are intermingled without clear spatial organization, a phenomenon also noted in earlier research by investigators including Dr. Clay Reid. The absence of spatial order suggests that neural connectivity in mice may depend on more selective and complex principles than simple proximity. It also raises the possibility that current imaging methods used in primates may be too coarse to detect more subtle patterns of organization. Understanding these interspecies differences has become a key area of investigation, with researchers now applying similar connectomic tools to study the monkey visual cortex.
“MICrONS represents the future,” says Dr. Andreas Tolias. “It is a foundational step toward building comprehensive brain models that integrate behavior, neural activity, and molecular biology.”
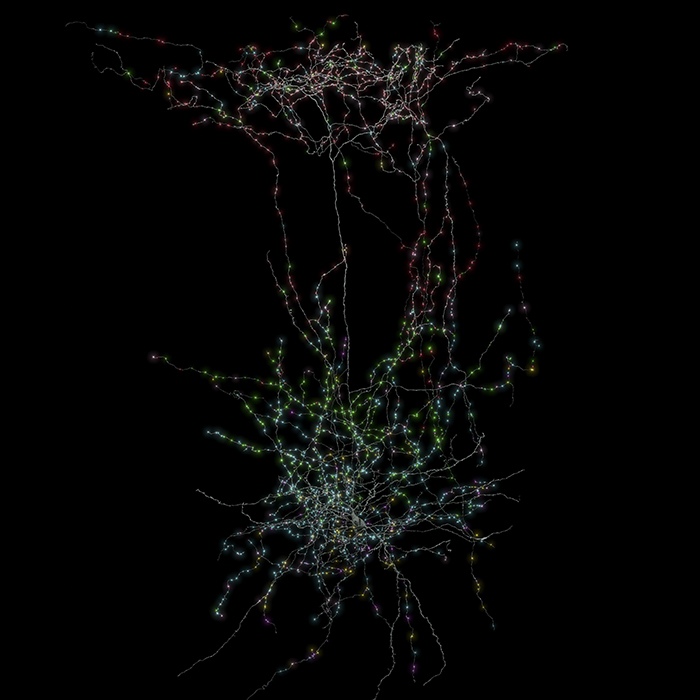
From Blueprint to Breakthroughs: Implications
The influence of the MICrONS project reaches far beyond the boundaries of basic neuroscience. By capturing both the structure and function of neural circuits, the project offers a powerful navigational tool that has been compared to a detailed map of the brain. “If you have a broken radio and a circuit diagram, you are better positioned to fix it,” says Dr. Nuno da Costa of the Allen Institute. “We can now compare the wiring of a healthy brain with that of disease models, which may help advance treatments for conditions such as Alzheimer’s, Parkinson’s, autism, and schizophrenia, where communication between neurons is disrupted.”
In addition to its medical implications, this extensive dataset holds great promise for the field of artificial intelligence. It offers valuable insights that could inspire new computational architectures modeled on the brain’s exceptional efficiency. These advances may influence future developments in areas such as robotics and natural language understanding.
Because the data is freely available to researchers around the world, it promotes international collaboration and accelerates discovery across disciplines.
The Power of Collective Science
MICrONS stands as a powerful testament to the strength of large-scale collaborative science. Funded primarily by the Intelligence Advanced Research Projects Activity (IARPA) and the National Institutes of Health through the BRAIN Initiative, the project brought together experts from a wide range of disciplines. At the center of the effort to coordinate the intricate electron microscopy work is Dr. Forrest Collman, whose multidisciplinary expertise has been essential in managing the enormous demands of the petabyte-scale computational infrastructure. “This kind of ambitious science depends on collaboration and a shared commitment to solving what once seemed impossible,” Collman says. “That is how progress is made.”
Dr. John Ngai, director of the BRAIN Initiative, highlights the project’s essential role: “Fundamental science like brain wiring maps lays the groundwork for understanding injury and disease. It brings us closer to real solutions for patients in need.”
Threshold of Discovery: From Impossible to Inevitable
MICrONS represents more than a remarkable feat of technology. It stands as a powerful reflection of the scientific spirit that dares to question boundaries once thought absolute. While the map produced so far captures only a tiny fraction of a mouse brain, it provides a crucial foundation for expanding these methods in future studies. Within this digital reconstruction lies an extraordinary scientific achievement, offering a vital step toward understanding the biological roots of thought and emotion.
The project also opens the door to deeper exploration of some of neuroscience’s most profound questions. It invites new inquiry into the differences between sensation and perception, and even into the elusive nature of consciousness. When asked whether such a map can shed light on consciousness, Dr. Forrest Collman offers a car metaphor to frame the discussion. He explains that the experience of driving arises from the coordinated function of the entire vehicle rather than any single part, such as the wheels or the steering mechanism. In much the same way, he suggests, consciousness emerges from the brain’s systems working in unison. “It is too complex and too much of an emergent phenomenon to be pinpointed in a detailed way,” he notes. While a wiring diagram alone may not uncover consciousness directly, it does offer a framework for asking more precise questions. These might focus on how the brain becomes aware of specific stimuli rather than attempting to define the entire phenomenon at once.
This distinction between intelligence and awareness remains one of the most intriguing frontiers in science, echoing philosophical themes found in fiction such as Peter Watts’ Blindsight. It is a space where neuroscience and philosophy continue to intersect, raising questions that challenge the limits of both disciplines.
As the field of neuroscience advances at an accelerating pace, MICrONS transforms ideas that once belonged to science fiction into grounded scientific discovery. From a tiny fragment of mouse brain, glowing as it responded to visual input, we catch a breathtaking glimpse of the mind’s intricate design. It is a beginning, one that lays the groundwork for countless discoveries still to come.
This article draws from research published in a collection of ten studies in the Nature family of journals in April 2025 and includes commentary from members of the MICrONS research team.