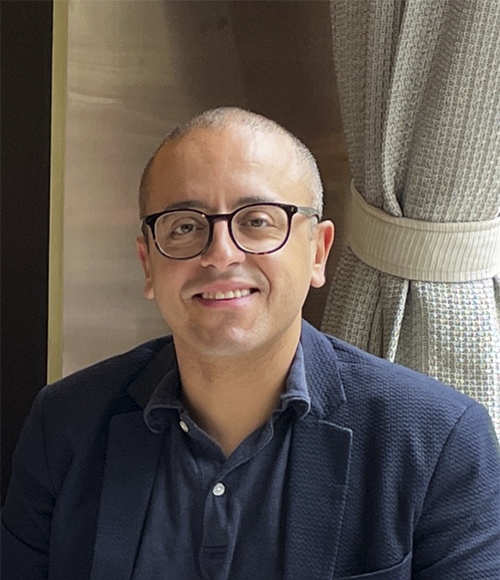
The Nuclear Envelope’s “Spiderweb” Mediating DNA Repair: Ground-breaking Research and its Implications for Cancer Therapy from Dr. Karim Mekhail and his Team
Researchers at the University of Toronto, led by Dr. Karim Mekhail, have made significant strides in understanding DNA repair mechanisms, potentially revolutionizing treatments for cancer and premature aging. Their study, published in Nature Structural and Molecular Biology, explores the nuclear envelope’s role in DNA repair, challenging previous assumptions about DNA mobility in mammalian cells.
Traditionally, processes mediating DNA repair are localized within the nucleus. However, Dr. Mekhail’s team discovered that when DNA is damaged, microtubule filaments from the cytoplasm exert pressure on the nuclear envelope, creating tubular structures or invaginations. These structures form a type of web that interacts with damaged DNA sites throughout the nucleus, significantly enhancing repair efficiency. This process can also be likened to how children push on balloons, creating indentations without popping them. The findings reveal the nuclear envelope as a dynamic mediator of DNA repair, transforming our perspective of the envelope from a passive boundary to an active player in maintaining genetic stability.
This discovery provides new insights into the dynamic responses of the nuclear architecture to DNA damage and opens potential pathways for therapeutic intervention. In conditions like progeria, a rare genetic disorder that accelerates aging, an overabundance of these nuclear tubules might compromise DNA repair. The study suggests that mutant lamin A, linked to progeria, induces these tubules, potentially affecting the cell’s ability to repair DNA. This work underscores the importance of cross-disciplinary collaborations, with Dr. Mekhail crediting his team’s diverse expertise for these advances.
The research further delves into gene knockdowns to identify which genes are essential for forming and dismantling the temporary envelope structures during DNA repair. By targeting and eliminating these nuclear envelope tubular structures, called dsbNETs, the team significantly reduced the tumor-forming capabilities of triple-negative breast cancer cells carrying BRCA1 mutations in mice. Although these results are promising, further research is needed to translate these findings into clinical applications.
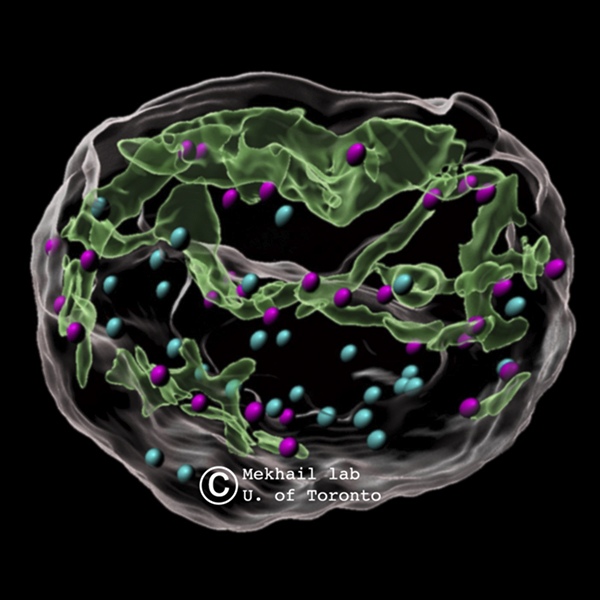
Motor proteins and microtubule filaments are crucial in this DNA repair process. Motor proteins generate the necessary movement to maneuver microtubule filaments within the cell, similar to how a child’s fingers push on a balloon. This force creates invaginations in the nuclear envelope, crucial for DNA repair. The study focused on kinesin motor proteins KIF5B and KIF13B, which push on the nuclear envelope to create dsbNETs. Another motor protein, KIFC3, moves in the opposite direction, reversing the structures formed during DNA repair and ensuring that cellular structures return to their normal state.
These findings have significant implications for cancer treatment. By manipulating dsbNET structures—either enhancing or suppressing them—researchers can develop strategies to target and control cancer cell growth selectively. This approach could also benefit diseases associated with premature aging, where similar DNA damage and repair mechanisms are involved. The ultimate goal is personalized medicine, tailoring treatments to each patient’s specific characteristics. While detailed customization may not yet be fully achievable, adapting these strategies to different cancer types should be feasible and could have a significant impact.
One of the most exciting and fundamental aspects of Dr. Mekhail’s research is the concept of reversible metamorphosis of the nucleus during DNA repair. Motor proteins and microtubule filaments drive this process, reshaping the nuclear envelope to facilitate DNA repair and then reverting it to its original state once the repair is complete. This dynamic ability maintains cellular integrity while effectively managing DNA repair.
Additionally, the study sheds light on how PARP inhibitors work in cancer treatment. Typically, cancers with BRCA1 and BRCA2 mutations rely heavily on PARP for DNA repair. PARP inhibitors prevent these cancer cells from repairing DNA damage, leading to cell death. The formation of dsbNETs increases the effectiveness of PARP inhibitors by facilitating incorrect repairs and chromosomal fusions, which are detrimental to cancer cells. Manipulating these structures can significantly influence cancer cell response to PARP inhibitors, offering potential strategies to boost treatment efficacy.
The research also suggests that elevated microtubule activity and unique nuclear structures could serve as biomarkers for certain cancers. By understanding and identifying these nuclear structures, pathologists and oncologists could diagnose and tailor treatments more effectively. This approach may enhance personalized medicine, enabling more accurate predictions of which cancers will likely respond to specific therapies, thus improving treatment outcomes.
Dr. Mekhail’s team is further dissecting the molecular mechanisms that govern the formation and reversal of dsbNETs while exploring therapeutic avenues based on modulating these structures. Building on his team’s diverse expertise, Dr. Mekhail aims to transform these molecular insights into practical therapies.
The primary focus of their research includes breast and ovarian cancers due to their prevalence and critical insights into cancer biology. Additionally, they are investigating disorders related to premature aging, such as Hutchinson-Gilford Progeria Syndrome (HGPS). This condition dramatically accelerates the aging process in children, and Dr. Mekhail’s continued research aims to improve outcomes for those affected while enhancing our understanding of natural aging processes.
Translating these discoveries into clinical applications is a long and complex journey involving rigorous testing, regulatory reviews, and obtaining necessary approvals. Dr. Mekhail’s research contributes to a broader understanding of cancer and aging at a molecular level, paving the way for novel therapeutic strategies that could revolutionize how we treat these conditions. The ultimate goal is to transform molecular insights into tangible treatments that improve patient outcomes, especially for those facing difficult-to-treat cancers and other severe diseases. This work underscores the importance of interdisciplinary collaboration and the dedication of the global scientific community to advancing biomedical science and improving human health.
Research article link:
Shokrollahi, M., Stanic, M., Hundal, A. et al. DNA double-strand break–capturing nuclear envelope tubules drive DNA repair. Nat Struct Mol Biol (17 April 2024). https://doi.org/10.1038/s41594-024-01286-7