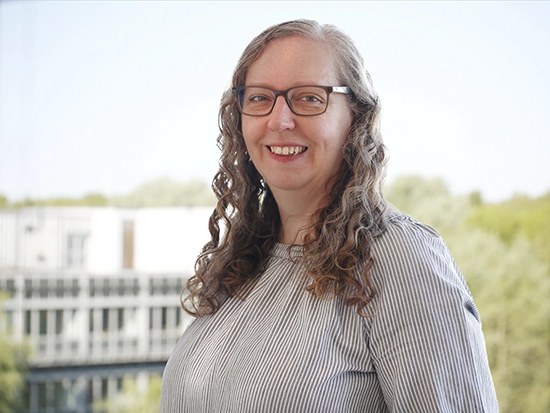
Uncovering a New Marine Symbiosis: Rhizobia and Diatoms Partner to Fix Nitrogen and Boost Marine Productivity
In a groundbreaking discovery, scientists have revealed that Rhizobia-like bacteria (Ca. Tectiglobus diatomicola), traditionally known for their symbiotic role in nitrogen fixation in legumes, also form partnerships with marine diatoms to fix nitrogen. This finding, detailed in a recent Nature publication by researchers from the Max Planck Institute for Marine Microbiology, the Alfred Wegener Institute, and the University of Vienna, solves a long-standing marine mystery and opens new avenues for understanding oceanic nitrogen cycles. Beyond marine biology, this discovery holds promise for revolutionary agricultural technologies and evolutionary insights, highlighting the critical role these bacteria play in marine productivity and carbon dioxide uptake.
Nitrogen is a fundamental component of life, essential for the growth of both terrestrial crops and marine plants, which contribute significantly to the planet’s oxygen production. While legumes on land have long been known to rely on Rhizobial bacteria to convert atmospheric nitrogen into a usable form, the mechanisms behind nitrogen fixation in the ocean have been less understood. The discovery that Rhizobia also engage in symbiosis with marine diatoms to fix nitrogen not only enhances our understanding of marine ecosystems but also bridges a crucial knowledge gap between terrestrial and marine nitrogen cycles.
This novel symbiotic relationship has far-reaching implications. The ability of marine Rhizobia-like bacteria to fix nitrogen and exchange it with diatoms in return for carbon positions them as key players in oceanic nitrogen fixation, particularly in regions where other nitrogen-fixing organisms are scarce. This discovery sheds light on the complex dynamics of marine ecosystems and hints at potential future applications in agriculture. The evolutionary traits of these marine symbionts might one day be harnessed to engineer nitrogen-fixing plants, potentially revolutionizing crop production and sustainability. In light of these exciting findings, we had the opportunity to interview Wiebke Mohr, one of the leading scientists on the study, to delve deeper into the implications of this research.
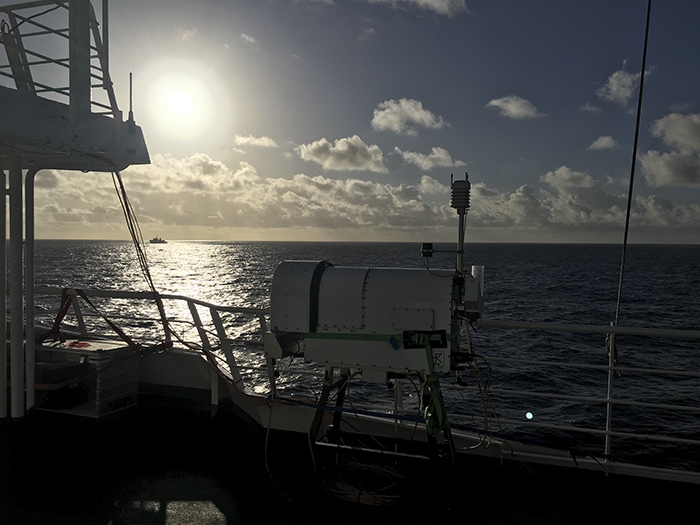
Q: Could you walk us through the initial stages of this research? What led you to explore the possibility of a symbiotic relationship between diatoms and Rhizobia-like bacteria?
A: When we look at the global perspective, nitrogen-fixing microorganisms in the ocean are just as important as those on land in terms of productivity. The ocean’s nitrogen fixation process is vital for maintaining ocean productivity because, like on land, the ocean is continuously losing nitrogen through various processes. To sustain productivity, nitrogen must be replenished, and that’s where nitrogen-fixing microorganisms play a crucial role.
Previously, it was assumed that most nitrogen fixation in the oceans was carried out by photosynthetic organisms called cyanobacteria. However, in vast regions of the ocean, the number of cyanobacteria present wasn’t sufficient to account for the measured nitrogen fixation. This led many scientists to hypothesize that non-cyanobacterial microorganisms might be responsible for the “missing” nitrogen fixation.
For about 25 years, we knew these organisms were in the ocean, but we didn’t know their true identity because they were only recognized by a partial sequence of their genes that code for the nitrogen-fixing enzyme. These genes can be transferred between bacteria, making it difficult to determine the organism’s identity just by the gene alone. Up until this point, the only organisms we had found actively contributing to nitrogen fixation on a global or regional scale were cyanobacteria either free-living or symbiotic.
Our interest in identifying the organisms responsible for this process stems from a need to understand where these microorganisms occur and why they are active in certain regions. This knowledge could help us predict how nitrogen fixation might change in the future, especially considering the challenges our planet faces with climate change. Identifying the specific organisms involved is key to understanding these potential changes, making this research both important and inherently fascinating.
Q: Could you describe the 2020 expedition to the North Atlantic and explain how it led to the discovery of the symbiotic relationship between diatoms and Rhizobia-like bacteria?
A: In 2020, my team and I traveled from Bremen to the tropical North Atlantic to join an expedition involving two German research vessels. We collected hundreds of liters of seawater from this region, to identify and quantify the importance of the mysterious nitrogen fixers.
Our expedition aimed to identify the non-cyanobacteria. One key approach we used was to collect the microorganisms not all together, but by different sizes. This method eventually led us to the bacteria we were searching for but it took us a couple of years to finally piece together its genome. Interestingly, we weren’t initially looking for symbiosis; we were simply trying to identify these organisms. However, during our research, we stumbled upon the symbiotic relationship between the diatoms and Rhizobia-like bacteria.
Q: The discovery was made around the Atlantic Ocean. Does this mean the organism is only found in localized areas, or can it be found globally?
A: Yes, our discovery was made in a specific region just east of the Caribbean Sea. We selected this area because historical measurements and previous research showed that nitrogen fixation rates are relatively high there compared to more open ocean waters, where the rates tend to be lower. In fact, the tropical and subtropical North Atlantic regions are particularly important, contributing about 20% of the global marine nitrogen fixation rate. We believed that by going to a region with high nitrogen fixation rates, we might have a better chance of finding these organisms. However, sequencing data from other regions of the ocean indicate that the newly found symbiont is globally distributed, and likely plays a significant role throughout other regions as well.
Q: Have you been collecting water samples from different areas to analyze the gene components in those samples?
A: Yes, exactly. Previous expeditions and other scientific groups on similar missions have been trying to identify these organisms in different areas of the oceans by either recovering a genome that contains not only the genes for the nitrogenase enzyme but also other taxonomic information that could help identify these organisms with a focus on finding the those that are actively fixing nitrogen in their natural environment. And we will continue to do so in the future.
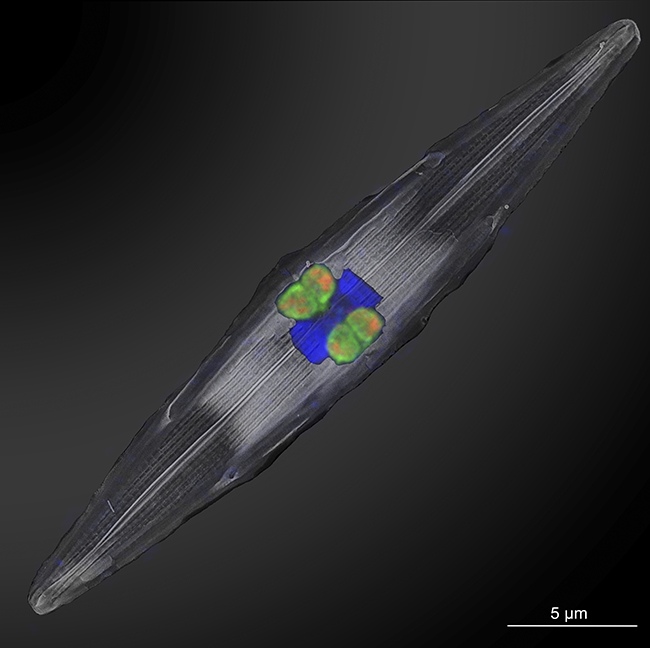
Q: What were some of the key challenges you encountered while identifying and characterizing the Rhizobia-like bacterium, Ca. Tectiglobus diatomicola?
A: One of the main challenges was the low abundance of these organisms. When you collect a liter of seawater, most of the microorganisms present are not nitrogen fixers; they belong to other groups. So when we sequence all the DNA from that liter of seawater, the majority of it belongs to non-nitrogen-fixing organisms. The nitrogen fixers, like the Rhizobia-like bacterium, are part of what we call the “rare biosphere”—they’re important, but they exist in very low numbers, making them hard to find.
To identify these rare organisms, we needed to collect a large volume of water and perform deep sequencing, which wasn’t as feasible 10-15 years ago due to the limitations in sequencing technology. Advances in sequencing have greatly improved our ability to detect these low-abundance organisms.
Another approach that helped us was separating the microorganisms by size. Instead of collecting everything together, we sorted them into different size fractions. This method allowed us to selectively enrich one organism in a fraction by sorting out others. It was somewhat of a lucky break that the symbiotic organisms ended up in these larger size fractions, which had fewer other organisms. So, it was a combination of improved sequencing technology, innovative approaches, and a bit of luck that allowed us to identify and characterize Ca. Tectiglobus diatomicola.
Q: How did the use of nanoSIMS technology enhance your understanding of the nitrogen exchange between the Rhizobia and diatom?
A: NanoSIMS technology is unique in its ability to definitively prove that an organism is actively fixing nitrogen during an incubation period with stable isotopes. It shows the actual integration of the stable isotope into the cellular biomass, allowing us to pinpoint nitrogen fixation activity down to individual microorganisms. Most other techniques lack the resolution to distinguish between individual organisms; they typically measure the biomass of a larger volume, like a liter of seawater, without being able to isolate the activity of specific cells.
NanoSIMS not only allows us to see this activity at the single-cell level but also helps us understand the nitrogen transfer to the diatom. Since no eukaryote, including diatoms, has been found to fix nitrogen on its own, the only way the diatom could obtain the enriched nitrogen is from its symbiont. Through the nanoSIMS imaging, we can confidently say that the diatom received this nitrogen from its symbiont. NanoSIMS technology thus enables us to precisely identify these activities and interactions at the microbial level, something other technologies can’t achieve.
This technology is relatively new, with the first applications to environmental microbiology emerging about 15 years ago. Our department was among the first to apply NanoSIMS in this field, marking a significant advancement in how we study microbial interactions and environmental processes.
Q: Your discovery suggests that this symbiosis plays a significant role in global marine nitrogen fixation. How do you see this affecting our current models of marine nitrogen cycles?
A: When we start to understand which organisms are responsible for a specific process—in this case, nitrogen fixation—and how they are distributed in the ocean, it will allow us to better understand what controls their activity. This could be influenced by factors like phosphorus-containing nutrients, iron-containing nutrients, temperature, or salinity. Ultimately, this knowledge will enable us to better predict how nitrogen fixation will develop in the future and improve the accuracy of models that inform us about the future state of our planet.
We’re still trying to understand what drives the activity of N2-fixing microorganisms—whether it’s phosphorus, iron, ocean currents, or other factors (and most likely a combination of them). We’re getting closer to understanding this as a scientific community, but we’re not there yet.
Q: Just out of curiosity, does nitrogen fixation produce more oxygen for the ocean?
A: It depends. There are two ways to look at it. In the short term, iIf the microorganism that fixes nitrogen (N2) is also a photosynthetic organism, like some cyanobacteria, then it would produce oxygen as well. If it’s in symbiosis with an algae—like our newly discovered organism, where the bacterium fixes nitrogen and the host diatom fixes carbon dioxide—then it does also contribute to oxygen production. However, there are also nitrogen-fixing organisms that don’t fix carbon themselves or don’t live in association with carbon-fixing phytoplankton, and these would not produce oxygen.
Therefore it’s important to understand what these organisms are and how they live, as it affects to what extent carbon dioxide can be drawn from the atmosphere.
In the long term, adding nitrogen to the water could potentially allow more carbon dioxide to be fixed, which could have broader implications for oxygen production and carbon cycling.
Q: What are the broader implications of this symbiotic relationship for marine productivity and carbon dioxide uptake?
A: In this case, the entire symbiotic relationship—where the symbiont and the host together are fixing both nitrogen (N2) and carbon dioxide (CO2)—plays a significant role. This combined ability allows them to take up carbon dioxide from the atmosphere and potentially contribute substantially to exporting carbon to the deep ocean over long timescales. Whether a nitrogen-fixer is also fixing CO2, either in symbiosis or alone, influences how much carbon dioxide – in the form of organic matter – can be delivered into the deep ocean.
So far, our research has focused on the Atlantic, but it will be interesting to see the impact, abundance, and activity of this symbiosis in other ocean basins. I’m sure our group, along with others, will be looking into this in different regions to understand its broader implications.
Q: You mentioned the evolutionary parallels between Ca. T. diatomicola and cyanobacterial endosymbionts like UCYN-A. Could you elaborate on the evolutionary significance of this discovery?
A: Until now, the majority of known symbiotic nitrogen-fixing microorganisms in the open ocean were cyanobacteria, such as UCYN-A and filamentous organisms like Richelia. The latter organisms have the ability to live symbiotically with diatoms, but they are all within the cyanobacteria group.
However, the discovery of a non-cyanobacterial symbiont like Ca. T. diatomicola suggests that the ability to enter into symbiosis with phytoplankton or algae developed independently in different groups. This opens up the possibility of finding even more types of these associations, whether they are as tight as this one or somewhat looser.
From an ecological perspective, this discovery could suggest greater resilience in marine ecosystems. If multiple types of organisms can form these symbiotic relationships, it could provide a buffer against environmental changes. For example, if one group can no longer tolerate increasing ocean acidity or temperature changes, another group might still be able to fulfill that role. Such functional redundancy could help balance the ecosystem if one organism loses its capacity to thrive.
Q: Do you believe that the relationship between Ca. T. diatomicola and diatoms could be an early-stage example of organelle formation? If so, what are the potential evolutionary paths this symbiosis might take?
A: At this point, we can only speculate, as we don’t have concrete confirmation. However, there are some intriguing signs. For instance, the genome of Ca. T. diatomicola is relatively reduced compared to its relatives, which is often seen in tight symbiotic relationships. Additionally, when we observe them under the microscope, we frequently notice that both the host and the symbiont seem to divide simultaneously. This coordinated cell division suggests that the symbiosis is very close, as the diatom needs to ensure that its symbiont is passed on to its offspring.
These observations could indicate that this relationship is a precursor to organelle form ation. The fact that both Ca. T. diatomicola and similar, cyanobacterial symbionts like the ones related to nitroplasts might be in similar stages—albeit developed independently—could provide insight into how these symbioses evolved. Whether they are more or less advanced, understanding these relationships will help us learn more about why these symbioses exist in their current forms and how they might evolve in the future.
Q: How do you envision this discovery influencing future agricultural practices, particularly in the context of engineering nitrogen-fixing plants? Given the small size and organelle-like nature of the marine Rhizobia, what challenges and opportunities do you foresee in potentially harnessing this bacterium for agricultural purposes?
A: This discovery opens up intriguing possibilities for agricultural practices, particularly in engineering nitrogen-fixing plants. The fact that the organism belongs to the Rhizobiales group is particularly interesting because plants are familiar with this group. Further, the small genome size of the diatom symbiont may facilitate the engineering of plants to have the genes to fix nitrogen themselves. While our research focuses on microorganisms, I hope plant researchers will explore these possibilities.
Many questions remain, and we’re just at the beginning of understanding this organism and its symbiotic relationships, but the possibilities are exciting.
Q: What are the next steps in your research? Are you exploring similar symbiotic relationships in other marine microorganisms?
A: There are two main aspects to our next steps. First, we want to try cultivating this organism, likely together with its host. We’re working on seeing if we can get them to grow in the lab, which is challenging because it involves growing two organisms together. However, there have been successes with similar symbioses, so we’re hopeful it can be done.
Secondly, we know there are other non-cyanobacterial N2-fixers out in the ocean that we don’t know much about. We’re interested in seeing if there are similar symbiotic relationships, either with other diatoms or with other types of phytoplankton. We strongly believe that other symbioses like this one exist, and we’re actively searching for them to identify and study them further.
Q: In your previous answer, you mentioned cultivating the organisms in the lab. Do you have to recreate the same environmental conditions, like the currents and temperature, to simulate their natural habitat?
A: That’s a great question. The reality is that, at the moment, the vast majority of microorganisms from the environment can’t be cultivated in the lab. We can only grow a small percentage of them, which is why we often use nanoscale approaches to study their activity in their natural settings. While we hope to cultivate these organisms, there’s no guarantee we’ll be successful.
To increase our chances, we’ll try to mimic their natural environment as closely as possible. This means providing a nutrient-poor environment, particularly low in nitrogen (like nitrate and ammonium), while ensuring they have all the other essentials they need—sufficient phosphate, iron, and light. We’ll likely try to grow them at temperatures around 25°C, maybe a bit higher. Essentially, we’re trying to create conditions that are ideal for them but not for other organisms, making it a “cozy” environment just for them.
Q: What do you hope the broader scientific community and the public take away from your research on this newly discovered symbiosis?
A: There are two key points I’d like to highlight. First, I hope others are as excited about this discovery as we are. Beyond the specific details, one broader takeaway is that as we continue to learn about microorganisms in the ocean, we often find that marine environments aren’t as different from terrestrial environments as we might think. This realization suggests that researchers studying land and ocean ecosystems can learn a lot from each other. For example, we never expected to find a Rhizobia-like microorganism in phytoplankton, but in hindsight, it makes sense because Rhizobia are common in terrestrial plants. I hope this serves as a spark for greater collaboration and knowledge-sharing between researchers working on land and those focusing on marine and freshwater environments.
On an even broader level, I hope this research reminds people that there are still countless discoveries to be made, even at the microbial level. Ocean research has gained a lot of public attention in the past five to ten years, especially regarding its importance for climate, health, and our overall well-being. Sometimes it might feel like we already know everything, but there are still many unknowns and surprises waiting to be uncovered. I hope our work encourages the scientific community and the public to stay curious and recognize that there is always more to learn, often from unexpected places.
Conclusion:
The discovery that Rhizobia bacteria form symbiotic relationships with marine diatoms marks a significant advancement in our understanding of nitrogen cycles in both marine and terrestrial environments. This finding not only solves a marine mystery but also opens doors to potential agricultural innovations. By bridging land and ocean ecosystems, this research highlights the interconnectedness of life on Earth and the potential of natural solutions to address global challenges. As exploration of these symbiotic relationships continues, we may see transformative impacts on food production and sustainability.